
Scotland’s Biodiversity Progress to 2020 Aichi Targets – Aichi Target 13 – Genetic Diversity Maintained – Supplementary Report 2020
Working with partners from SEFARI, we have developed a world first genetic diversity indicator for wild species. We use a scorecard approach for wild species of cultural and socio-economic importance to promote long-term conservation of genetic diversity and address Aichi Biodiversity Target 13.
Of the 26 species assessed in this report:
- 14 species were classed as being at negligible genetic risk;
- Eight species were classed as being at moderate risk, with effective mitigation in place for five of these;
- Four species were classed as being at risk of severe genetic problems.
See also Scotland's Biodiversity Progress to 2020 Aichi Targets – Aichi Biodiversity Target 13 method report.
Acknowledgements
Funding – we acknowledge funding from SEFARI Gateway ‘Think Tank’ scheme, the Natural Environment Research Council, and the Scottish Government Rural and Environment Science and Analytical Services (RESAS) division.
Photography – all images by Lorne Gill/SNH other than Gordon Rothero p. 8.; RBGE p. 18, 26, 34 and 36; Sarah Lambert p. 38; Dave Genney/SNH p. 40 and 42; Sue Scott/SNH p. 44; David Whitaker p. 48; Laurie Campbell/SNH p. 64.
Data – we are grateful to the Botanical Society of Britain and Ireland, the National Biodiversity Network (NBN) and SNH for distributional data, and the Millennium Seed Bank at Royal Botanic Garden Kew for information on their holdings.
Maps – contain Ordnance Survey data © Crown copyright and database right (2019).
AICHI TARGET 13 – GENETIC DIVERSITY MAINTAINED
This report covers wild species of socio-economic importance. It complements the assessment of cultivated plants, and farmed and domesticated animals, and their wild relatives, which can be found in the main body of the report.
Methodology
As there is no agreed national list of species of socio-economic and/or cultural value for Scotland, we developed a set of criteria for selecting species. These include:
- Species prioritised for conservation value
- Species identified as being culturally important
- Species providing important ecosystem services
- Game species
- Species collected for food or medicine
Using these criteria, we selected 26 species for initial assessment. For each species, we produced a Genetic Scorecard, outlining:
- Relevant genetic conservation issues for the species in question
- The importance of its genetic diversity on an international scale
- An evaluation of the genetic risks facing in situ populations
- A statement of confidence in the assessment
- The degree to which representation in ex situ collections mitigate against genetic diversity loss (further explanation of these maps is provided in Section 4).
- An overall ‘traffic light’ score of genetic risks and whether current conservation actions are effective
Using 2010 as a baseline reference point, the approach assesses contemporary genetic issues, and likely future issues during a 25-year window from the point of assessment.
For quantification of levels of risk, we adopted the following framework:
- Negligible: No obviously detectable genetic problems occurring or expected over the next 25 years.
- Moderate: Moderate genetic problems occurring or expected over the next 25 years; e.g.:
- Moderate loss of populations that are likely to contain unique diversity (e.g., resulting in losses of up to 25% of important genetic types / distinct populations);
- Clearly observable fitness problems in up to 25% of populations due to low genetic variation and subsequent inbreeding depression;
- Marked and clearly observable loss of genetic integrity by hybridisation at up to 25% of populations;
- Severe restrictions on regeneration/recruitment/reproduction in many or most populations of long-lived species limiting evolutionary change in the immediate future.
- Serious: Serious genetic problems occurring or expected over the next 25 years; e.g.:
- Severe loss of populations that are likely to contain unique diversity (e.g., resulting in losses of > 25% of important genetic types / distinct populations);
- Loss of any highly divergent endemic lineages that are globally unique;
- Strong, clearly observable fitness problems in >25% of populations due to low genetic variation and subsequent inbreeding depression;
- Marked and clearly observable loss of genetic integrity by hybridisation at >25% of populations.
The assessment is based on expert opinion, using direct genetic data, where available, combined with information on species biology, abundance and distribution. Where no direct genetic data are available, the genetic risk assessment is based on species biology, abundance and distribution.
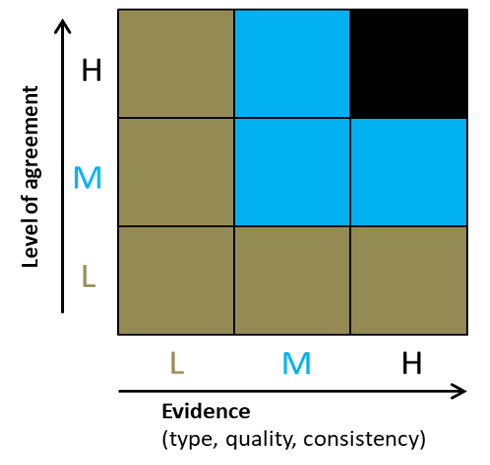
For quantification of uncertainty in the production of the genetic scorecards, we use the approach adopted for the UK Biodiversity Climate Change Impacts Report Card (Figure 1). This combines the level of agreement among experts and the amount and type of available evidence. The overall confidence level is governed by the lowest score for either expert agreement or evidence availability.
We classify whether current management actions are appropriate and effective for mitigating any current or imminent genetic problems. This is articulated via a 4-point scale:
- Mitigation not required
- The current status of the species is stable with negligible genetic risks
- Mitigation effective
- Although there is a risk to genetic diversity, current measures are considered to be effective and sufficient to improve the situation
- Mitigation not effective
- There is a recognised risk to genetic diversity and current mitigation are not considered to be effective or sufficient
- Mitigation not in place
- There is a recognised risk to genetic diversity and mitigation is not in place
Full details of the methodology are presented in Hollingsworth et al. (2019) Conserving Genetic Diversity: Development of a national approach for addressing Aichi Biodiversity Target 13 that includes wild species. SEFARI & SNH.
Scottish distribution maps presented in the Scorecards for each species use data from the
National Biodiversity Network, showing Scottish records since 1992 unless otherwise stated.
Summary of findings
Twenty-six species of socio-economic and/or cultural importance were assessed in this report, ranging from extremely rare species of high national conservation importance, through to widespread dominant species that provide important functional ecosystem services. Table 1 summarises the genetic risk scores for each species and the primary pressures associated with any genetic risks.
Of the 26 species assessed in this report:
- 14 species were classed as being at negligible genetic risk
- Eight species were classed as being at moderate risk, with effective mitigation in place for five of these
- Four species were classed as being at risk of severe genetic problems
Serious risk species
Of the four species classified as being at serious risk, two (Scottish wildcat and ash) are primarily threatened by non-native species. In the case of the Scottish wildcat, the primary threat is extinction-by-hybridisation from feral domestic cats, with the small number of remaining pure Scottish wildcats being vulnerable to further loss of genetic integrity. In the case of ash, although there are millions of ash trees still present in the UK, there is a serious risk of genetic diversity loss due to large-scale mortality from the introduced ash-dieback pathogen, and the potential for further pressures from the introduced emerald ash borer. For both Scottish wildcat and ash, despite extensive mitigating actions being undertaken, the scale of threat means that the risks to their genetic diversity remain serious.
In the case of the great-yellow bumblebee, land-use change leading to sub-optimal habitat management has resulted in population declines, and this, coupled with the short-life cycle of the species, creates a risk of further rapid decline in genetic diversity. Likewise, the freshwater pearl mussel is experiencing continued population declines with the associated risk of genetic diversity loss.
Moderate risk species
Many of the species at moderate risk of genetic problems are relatively widespread species facing acute pressures from pests/pathogens and/or non-native species. For instance, closely related non-native species are a source of pressure for both the British bluebell and the red squirrel, farmed salmon is a pressure for Atlantic salmon, and risks of population declines due to pests and pathogens are important for Scots pine, Atlantic salmon, sea trout/brown trout, red grouse and red squirrel. The primary threat to the woolly willow in contrast, is its rarity, occurring in just 12 populations, many with very few individuals, with only the longevity of individual bushes acting as a buffer against immediate serious genetic diversity loss.
Of the eight species classed as being at moderate risk, effective mitigation is not in place for sea trout/brown trout and Atlantic salmon, or for the Scottish bluebell.
Negligible risk species
Species scored as having low levels of genetic risk were often species that are common. Two of the categories (ecosystem service provision, food/medicine) were dominated by widespread species with negligible genetic risks, reflecting in part the criteria used for selection. For instance, species providing important ecosystem services were selected based on high levels of land cover. Likewise, the species of importance for food/medicine were selected based on reported high frequency of use by foragers. These selection criteria resulted in the inclusion of extremely common species in the assessment, and their abundance is in turn associated with negligible genetic risks (abundance acts as a considerable buffer against genetic diversity problems). However, it is noteworthy that risks of genetic diversity loss can still occur in abundant species, as illustrated by the serious risk score for ash.
- |
Selection criteria Conservation |
Selection criteria Culturally Important |
Selection criteria Ecosystem servces |
Selection criteria Food/medicines |
Selection Criteria Game |
Taxonomic group |
Risk and response |
Confidence
|
Types of threat associated with moderate/ serious genetic risks |
Page No. |
---|---|---|---|---|---|---|---|---|---|---|
Papillose bog-moss Sphagnum papillosum |
- |
- |
yes |
- |
- |
Bryophyte |
Negligible risk Mitigation not required |
Med |
n/a |
8 |
Scots pine Pinus sylvestris |
- |
yes |
- |
- |
- |
Vascular plant |
Moderate risk Mitigation effective |
High |
Pathogens, regeneration limitation |
10 |
Raspberry Rubus idaeus |
- |
- |
- |
yes |
- |
Vascular plant |
Negligible risk Mitigation not required |
High |
n/a |
12 |
Oak Quercus spp. |
- |
yes |
- |
- |
- |
Vascular plant |
Negligible risk Mitigation effective |
High |
n/a |
14 |
Woolly willow Salix lanata |
yes |
- |
- |
- |
- |
Vascular plant |
Moderate risk Mitigation effective |
High |
Small isolated populations, regeneration limitation |
16 |
Heather Calluna vulgaris |
- |
yes |
yes |
|
- |
Vascular plant |
Negligible risk Mitigation not required |
High |
n/a |
18 |
Blaeberry Vaccinium myrtillus |
- |
- |
- |
yes |
- |
Vascular plant |
Negligible risk Mitigation not required |
Med |
n/a |
20 |
British bluebell Hyacinthoides non-scripta |
- |
yes |
- |
- |
- |
Vascular plant |
Moderate risk Mitigation effective |
High |
Hybridisation & competition with non-native species |
22 |
Harebell/Scottish bluebell Campanula rotundifolia |
- |
yes |
- |
- |
- |
Vascular plant |
Moderate risk Mitigation not in place |
Med |
Population declines due to pollution |
26 |
European ash Fraxinus excelsior |
- |
- |
- |
- |
- |
Vascular plant |
Serious risk Mitigation not effective |
High |
Pests/pathogens |
30 |
Elderberry Sambucus nigra |
- |
- |
- |
yes |
- |
Vascular plant |
Negligible risk Mitigation not required |
Med |
n/a |
34 |
Yorkshire fog Holcus lanatus |
- |
- |
yes |
- |
- |
Vascular plant |
Negligible risk Mitigation not required |
Med |
n/a |
36 |
Purple moor-grass Molinia caerulea |
- |
- |
yes |
- |
- |
Vascular plant |
Negligible risk Mitigation not required |
Med |
n/a |
38 |
Hazel gloves Hypocreopsis rhododendri |
yes |
- |
- |
- |
- |
Fungus |
Negligible risk Mitigation effective |
High |
n/a |
40 |
Chanterelle Cantharellus cibarius |
- |
- |
- |
yes |
- |
Fungus |
Negligible risk Mitigation not required |
Med |
n/a |
42 |
Freshwater pearl mussel Margaritifera margaritifera |
yes |
- |
- |
- |
- |
Mollusc |
Serious risk Mitigation not effective |
Med |
Population declines due to pollution, exploitation and climate change |
44 |
Great yellow bumblebee Bombus distinguendus |
yes |
- |
- |
- |
- |
Insect |
Serious risk Mitigation not effective |
Low |
Population declines associated with habitat loss, climate change |
46 |
Sea trout/brown trout Salmo trutta |
- |
- |
- |
- |
yes |
Fish |
Moderate risk Mitigation not in place |
Low |
Pests/pathogens, hybridisation with nonnative species, climate change, pollution |
48 |
Atlantic salmon Salmo salar |
- |
yes |
- |
- |
yes |
Fish |
Moderate risk Mitigation not effective |
High |
Pest/parasites, hybridisation with farmed stock, climate change, pollution |
52 |
Common frog Rana temporaria |
- |
|
- |
yes |
- |
Amphibian |
Negligible risk Mitigation not required |
High |
n/a |
54 |
Golden eagle Aquila chrysaetos |
- |
yes |
- |
- |
- |
Bird |
Negligible risk Mitigation effective |
High |
n/a |
56 |
Red grouse Lagopus lagopus |
- |
|
- |
- |
yes |
Bird |
Moderate risk Mitigation effective |
High |
Pests/pathogens, population declines associated with habitat loss |
58 |
Red squirrel Sciurus vulgaris |
- |
yes |
- |
- |
- |
Mammal |
Moderate risk Mitigation effective |
Med |
Pests/pathogens, hybridisation & competition with non-native species |
60 |
Scottish wildcat Felis silvestris |
yes |
- |
- |
- |
- |
Mammal |
Serious risk Mitigation not effective |
High |
Hybridisation with non-native species |
62 |
Red deer Cervus elaphus |
- |
yes |
- |
- |
yes |
Mammal |
Negligible risk Mitigation not required |
High |
n/a |
64 |
Roe deer Capreolus capreolus |
- |
- |
- |
- |
yes |
Mammal |
Negligible risk Mitigation not required |
High |
n/a |
66 |
Species reports


|
- |
---|---|
Background |
Mat-forming widely distributed dioecious moss; the most common bog moss in cool-temperate Eurasia, and an indicator of good habitat quality due to low tolerance of pollution and shade (Blockeel et al., 2014). Also widespread in wet grassland, heath and other wet peaty habitats (Atherton et al., 2010). This single species is the main component of most peat deposits (Blockeel et al., 2014) and therefore crucial for carbon storage. It is uniformly polyploid across the circumboreal zone (Karlin et al., 2010). Globally, Sphagnum distribution is expected to contract due to climate warming (Bragazza, 2008; Gallego-Sala et al., 2013). |
Current threats |
Most bogs in the UK have been substantially modified by artificial drainage, afforestation, overgrazing, burning, erosion and pollution (Bragg & Tallis, 2001). Climate and management are predicted to result in increased erosion over parts of the UK (Li et al., 2016), and over 50% of bog habitats currently exceed nitrogen critical loads leading to preferential growth of more competitive grasses and direct toxicity (APIS, 2019a). |
Contribution of Scottish population to total species diversity |
No evidence for divergent genetic lineages being present in Scotland. |
Diversity loss: population declines |
Despite ongoing population declines, the overall abundance of the species suggests any imminent loss of genetic diversity will be negligible. |
Diversity loss: functional variation |
Acidification during the Industrial Revolution resulted in historical loss of S. papillosum from parts of England which may have involved past loss of adaptive variation (Blockeel et al., 2014). However, no direct evidence of historical losses from Scotland, and no overall evidence of loss of functional/adaptive variation. |
Diversity loss: divergent lineages |
Negligible risk (no evidence for divergent lineages in Scotland). |
Hybridisation/ introgression |
None known. |
Low turnover/ constraints on adaptive opportunities |
Turnover rates in these long-lived species are poorly known. Sexual reproduction is common and experiments in cultivation suggest spores are easy to obtain and grow (Gaudig et al., 2014). |
In situ genetic threat level |
Negligible (a very abundant and widespread species despite many ongoing risks from habitat loss and degradation). |
Confidence in in situ threat level |
Medium (assessment based on good distribution and ecological data supported by direct data on most aspects of species’ distribution and management requirements, but lacking direct genetic data). |
Ex situ representation |
Some material is held in ex situ cultivation for both micropropagation and experimental farming techniques (Caporn et al., 2018; Gaudig et al., 2014). No structured sampling to-date to represent S. papilosum in spore banks. |
Current conservation actions |
Raised bog and blanket bogs are conservation priority habitats under EU habitats directives (92/43/EEC) and UK government supports phasing out peat in cultivation. Restoration of peatlands is an ongoing activity, and although hydrologic conditions can successfully be restored, vegetation recovery depends on additional factors (topography and climate). |
Overall T13 status |
Negligible risk; Mitigation not required |
Overall T13 status explanation |
Limited immediate genetic concerns due to the shear abundance of the species. Pollution and climate change may, in the future, lead to population declines that create genetic problems, but in the immediate future the risk is low. |
Assessor |
Rebecca Yahr, Royal Botanic Garden Edinburgh |
Reviewer |
Aline Finger, Royal Botanic Garden Edinburgh Pete Hollingsworth, Royal Botanic Garden Edinburgh |
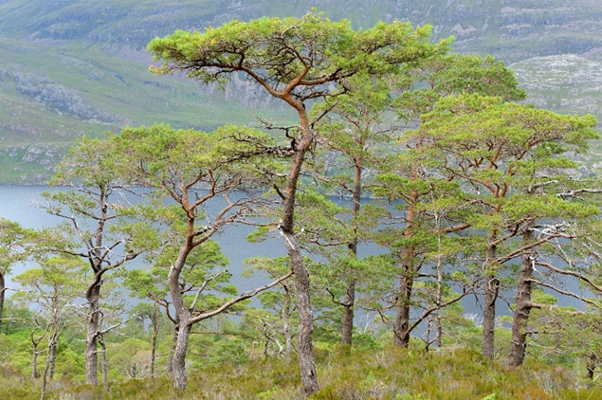
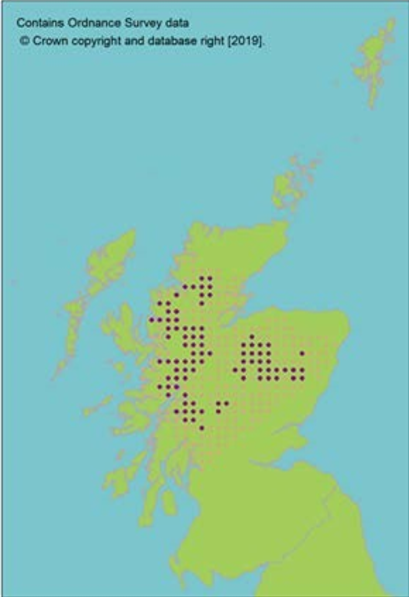
|
- |
---|---|
Background |
Hermaphrodite, wind pollinated, widely distributed tree. Present in 84 natural stands, often small and fragmented (dark circles on map, light circles are plantations). Natural stands represent only 10% of trees in Scotland. Genetic marker studies show large amounts of neutral genetic diversity. Some evidence of adaptive differentiation in Scotland from west to east (Salmela, 2011; Donnelly et al., 2018). |
Current threats |
Plant pathogens represent the major emerging threat (Dothistroma septosporum races introduced on Corsican and lodgepole pine) (Piotrowska et al., 2018). |
Contribution of Scottish population to total species diversity |
Molecular evidence for putative separate lineage in north western Scotland, although nuclear markers indicate very low differentiation, even from continental Europe (Ennos et al., 1997). Scotland does, however, contain a uniquely oceanic adapted population (Ennos et al., 1997; Donnelly et al., 2018). |
Diversity loss: population declines
|
Multiple small populations with no regeneration coupled with a biased age-structure towards older trees compromises the sustainability of many populations. However, there is limited risk of imminent genetic diversity loss due to high levels of standing variation in adult trees (assuming no catastrophic population losses due to pathogens). |
Diversity loss: functional variation
|
The general persistence of the species across its range in Scotland is not threatened, which minimises likely loss of adaptive variation. There are risks to loss of high elevation populations across its range which may lead to some loss of adaptive variation. |
Diversity loss: divergent lineages |
Limited divergence from European populations precludes loss of major divergent lineages. The most genetically distinct populations are in the north west of Scotland around Shieldaig. These populations are not currently threatened. |
Hybridisation/ introgression |
Buffer zones in which planting of non-local seed is prohibited around existing native stands limit risk to loss of integrity from exotic stands. |
Low turnover/ constraints on adaptive opportunities |
Deer grazing is a major limitation on turnover and regeneration, but the risk is mitigated in c. 20% of populations where active management is in place. |
In situ genetic threat level |
Moderate (in the face of emerging pathogen threats, major limitations to regeneration present a moderate risk of genetic variation loss and constraints to adaptation). |
Confidence in in situ threat level |
High (assessment based on good demographic data and direct data on genetic variation, population differentiation and biology). |
Ex situ representation |
Seeds from 13 10km squares held at the Millennium Seed Bank, including all 5 UK ‘standard’ tree seed zones in which native stands occur, with 68% ex situ coverage of its wild extent of occurrence. |
Current conservation actions |
Grazing controls at c. 20% of sites promote regeneration providing adaptive opportunities. Establishment of Gene Conservation Unit at Beinn Eighe National Nature Reserve safeguards some variation. |
Overall T13 status |
Moderate risk; Mitigation effective |
Overall T13 status explanation |
Despite the fragmented nature and small size of many populations, longevity of individual trees minimises imminent loss of genetic diversity. Management to promote regeneration supports some ongoing evolutionary processes, and wide representation of all seed zones in seed banks likely catches main adaptive variation. |
Assessor |
Richard Ennos, University of Edinburgh |
Reviewer |
Stephen Cavers, Centre for Ecology and Hydrology Peter Hollingsworth, Royal Botanic Garden Edinburgh |
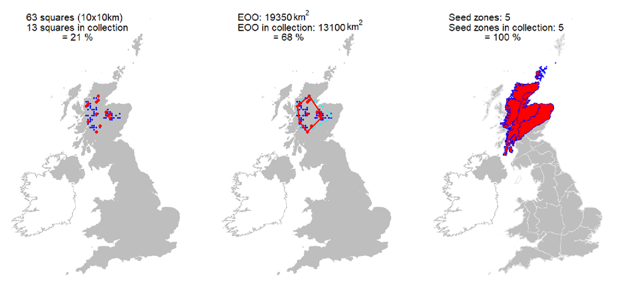
Seeds from 13 10km squares held at the Millennium Seed Bank, including all 5 UK ‘standard’ tree seed zones in which native stands occur, with 68% ex situ coverage of its wild extent of occurrence.


|
- |
---|---|
Background |
Widespread woody perennial reproducing via seed and suckering. Raspberry is native to Europe and Asia, and is found throughout Scotland, including the Northern Isles where the species is considered to be introduced. Raspberry is widely cultivated both as a commercial crop and for domestic use. |
Current threats |
Hybridisation has been recorded in the UK with other Rubus species. Introgression between domestic cultivars and wild raspberry has been recorded. Genetic diversity has been reduced in domesticated populations (Haskell, 1960; Graham & McNicol, 1995). In Tayside wild populations remain more diverse than cultivated plants (Graham, Marshall & Squire, 2003). |
Contribution of Scottish population to total species diversity |
With a wide distribution within temperate Europe and Asia, and predominantly sexual reproduction, the Scottish population of raspberry is likely to make a small contribution to the global species diversity. |
Diversity loss: population declines |
Scrub clearance is likely to have led to localised reductions in populations, but given the remaining abundance and distribution of the species, any diversity losses are likely to be minimal. |
Diversity loss: functional variation
|
There is some evidence for adaptive differences between populations (e.g., populations at high altitudes can have later budburst and shorter growth than other populations; Jennings, 1964). However, there is no evidence of declines that are leading to a marked loss of populations containing unique adaptive variants. |
Diversity loss: divergent lineages |
Considered negligible risk. |
Hybridisation/ introgression
|
Low risk. Hybridisation has been recorded between raspberry and other Rubus species, but although gene flow occurs, it is infrequent and localised (Lusby & McNicol, 1995). Despite several decades of potential exposure to commercial clones in Tayside, gene flow between wild populations and cultivars is low (Graham et al., 2003). |
Low turnover/ constraints on adaptive opportunities |
No evidence for constraints on reproduction. |
In situ genetic threat level |
Negligible (extremely widespread species, limited threat from hybridisation). |
Confidence in in situ threat level |
High (direct genetic data available, abundant species). |
Ex situ representation |
The Millennium Seed Bank has collections from 23 10 km squares, from widely distributed localities in the UK, including comprehensive coverage of Scotland. Many commercial cultivars grown in Scotland. |
Current conservation actions |
Promotion of pollinator-friendly management provides an opportunity for lowland populations of raspberry to increase. |
Overall T13 status |
Negligible risk: Mitigation not required |
Overall T13 status explanation |
The widespread distribution and low level of introgression with domestic cultivars indicate that the Scottish raspberry population is genetically healthy with no evidence for genetic problems. |
Assessor |
Iain Macdonald, Scottish Natural Heritage |
Reviewer |
Angus Hannah, BSBI Pete Hollingsworth, Royal Botanic Garden Edinburgh |

The Millennium Seed Bank has collections from 23 10 km squares, from widely distributed localities in the UK, including comprehensive coverage of Scotland. Many commercial cultivars grown in Scotland.
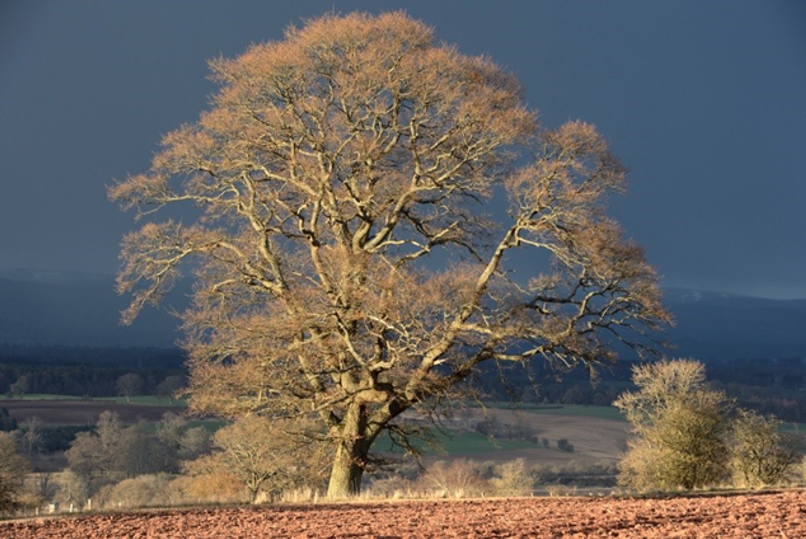

|
- |
---|---|
Background |
Hermaphrodite, wind-pollinated and bird-dispersed (jay, Garrulus glandarius), widely distributed tree. Present in both natural populations and in current and former plantations, the latter often established historically for charcoal and bark production under coppice management. In the west of Scotland, the oaks are keystone species within the Atlantic rainforests. The two species regularly hybridise in Scotland (Gerber et al., 2014). Genetic marker studies show colonisation exclusively from an Iberian refugium (Cottrell et al., 2002), and that populations retain large amounts of neutral genetic diversity at nuclear loci. There is evidence of adaptive differentiation in the UK on a north-south axis (Wilkinson et al., 2017). |
Current threats |
A syndrome of ill health in principally older oak populations in the UK has been dubbed ‘oak decline’, but this appears to be a problem associated with ageing trees located in inappropriate habitats. Many woodlands throughout Scotland lack regeneration due to high browsing pressure. |
Contribution of Scottish population to total species diversity |
Scottish populations share their postglacial origins with oaks in Spain and France and show little differentiation for neutral markers (Cottrell et al., 2002). Scottish populations show evidence of adaptation to shorter growing seasons compared to populations from elsewhere in the distribution. |
Diversity loss: population declines
|
There is limited risk of imminent genetic diversity loss due to high levels of standing variation in adult trees. However, if the problem of lack of natural regeneration is not solved long-term losses due to inability to adapt to future environments are likely. |
Diversity loss: functional variation |
The general persistence of the species complex across its range in Scotland is not threatened, which minimises likely loss of adaptive variation. |
Diversity loss: divergent lineages |
Limited divergence from European populations in Spain and France precludes loss of major divergent lineages. |
Hybridisation/ introgression
|
Hybridisation between the two oak species is a natural and common phenomenon in Scotland. Despite high levels of hybridisation, the integrity of the individual species remains. |
Low turnover/ constraints on adaptive opportunities |
Lack of regeneration in existing oakwoods has long been a problem. Overgrazing by deer and livestock is an important contributor. However, lack of recognition of the role of jays in facilitating regeneration in other areas of the landscape e.g. birchwoods means that grazing protection measures have often not been well targeted (Worrell et al., 2014). |
In situ genetic threat level |
Negligible (major limitations to regeneration pose no immediate threat, but may lead to loss of diversity in the long term if not addressed). |
Confidence in in situ threat level |
High (detailed genetic and demographic data available). |
Ex situ representation |
Oak is not currently represented in seed banks, as it is ‘recalcitrant’. Acorns are sensitive to desiccation, so they cannot be stored in conventional seed bank conditions of low humidity and temperature. A project at Kew is working to develop cryopreservation techniques and storage protocols to bank recalcitrant seeds. Our native oaks are represented in many botanic gardens and arboreta, including the Royal Botanic Gardens Edinburgh. |
Current conservation action |
The key management intervention is management to promote regeneration. |
Overall T13 status |
Negligible risk; Mitigation effective |
Overall T13 status explanation |
Longevity of individual trees minimises imminent loss of genetic diversity. Management to promote regeneration supports some ongoing evolutionary processes. Proactive work to understand potential impact from pathogens also underway. |
Assessor |
Richard Ennos, University of Edinburgh |
Reviewer |
Jeanette Hall, Scottish Natural Heritage Pete Hollingsworth, Royal Botanic Garden Edinburgh |
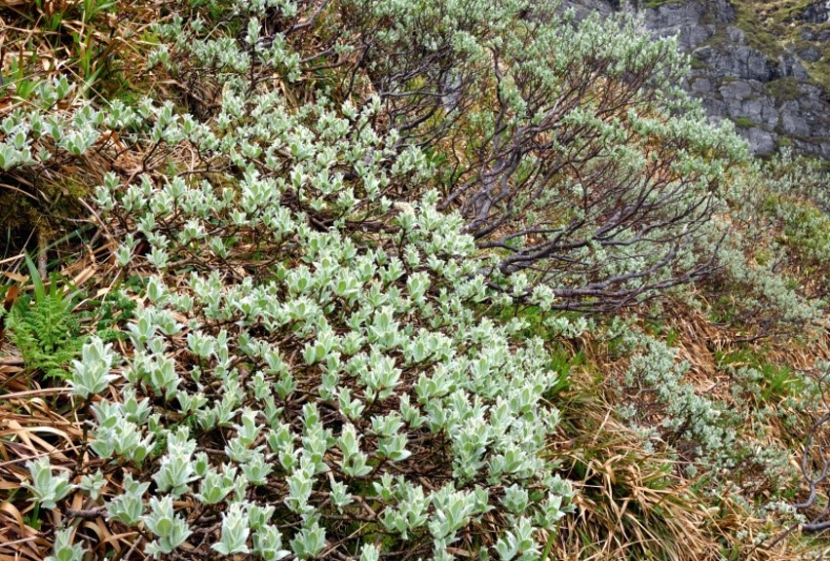

|
- |
---|---|
Background |
Perennial dioecious shrub occurring in 14 10 km squares in Scotland; only two sites exceed 200 individuals and three sites contain only a single sex. Capable of local clonal spread, but most individual bushes are unique genotypes consistent with a primarily sexual reproduction. Considered to be previously more widespread (Marriott et al., 2016). Population genetic studies show low population differentiation and little correlation between population size and genetic diversity consistent with the longevity of individual bushes acting to retard rates of genetic erosion in small populations (Scottish Montane Willow Group, 2005). |
Current threats |
Primary threat is grazing, restricting the species to steep crags and cliffs at most sites. |
Contribution of Scottish population to total species diversity |
The species is common elsewhere in its range and there is no expectation of divergent endemic genetic lineages in Scotland, although the populations may be adapted to the local environment. |
Diversity loss: population declines
|
Populations are small, fragmented and isolated but existing evidence shows low population differentiation and limited evidence for genetic diversity loss: individual plant longevity ameliorates short term risks. |
Diversity loss: functional variation |
No immediate risk to functional variation from population declines. Populations occupy relatively similar environmental conditions, minimising likelihood of unique adaptive variants. |
Diversity loss: divergent lineages |
Limited risk as no evidence for phylogenetically divergent lineages present in Scotland. |
Hybridisation/ introgression
|
The species co-exists with hybridising species, and inter-specific hybrids are recorded at several sites. However, genetic marker data give no evidence for extinction-by-hybridisation risk (Forrest, 2006). |
Low turnover/ constraints on adaptive opportunities |
Regeneration is considered a limiting factor due to grazing limiting flower production coupled with poor seedling establishment (Scottish Montane Willow Group, 2005). This will limit levels of adaptive change. |
In situ genetic threat level |
Moderate (small fragmented populations of hybridising species present genetic risks, mitigated by the longevity of individual plants). |
Confidence in in situ threat level |
High (assessment based on good demographic data and direct data on genetic variation, population differentiation and hybridisation). |
Ex situ representation |
Two accessions from one 10 km square held at the Millennium Seed Bank, resulting in partial coverage of Scotland; further collections being sourced. Additional representation in the Royal Botanic Garden Edinburgh living collection of 31 accessions from six Scottish populations. |
Current conservation actions |
Restoration underway at four sites, involving seed and cutting collection, ex situ propagation, and translocation to reinforce extant populations. Grazing controls in place at most important populations. |
Overall T13 status |
Moderate risk; Mitigation effective |
Overall T13 status explanation |
Despite the small population sizes and fragmented nature of the species range, conservation interventions have halted declines, there is representation in ex situ collections, and restoration interventions are increasing numbers of individuals in situ. |
Assessor |
Pete Hollingsworth, Royal Botanic Garden Edinburgh |
Reviewer |
Rob Ogden, University of Edinburgh |
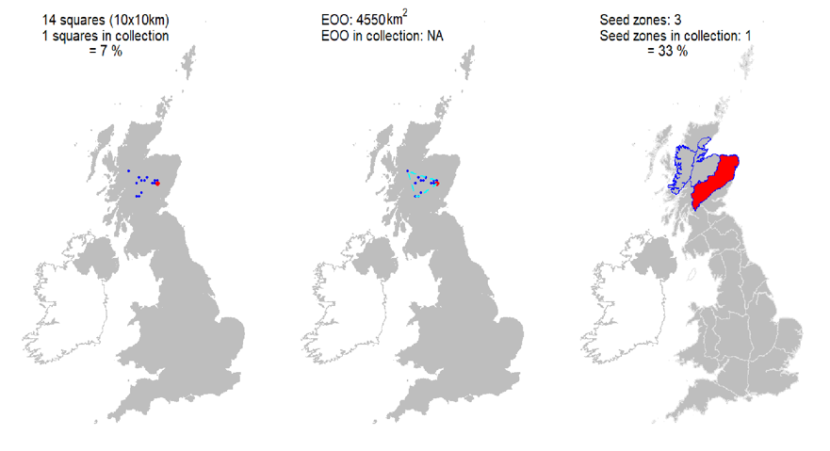
Two accessions from one 10 km square held at the Millennium Seed Bank, resulting in partial coverage of Scotland; further collections being sourced. Additional representation in the Royal Botanic Garden Edinburgh living collection of 31 accessions from six Scottish populations.
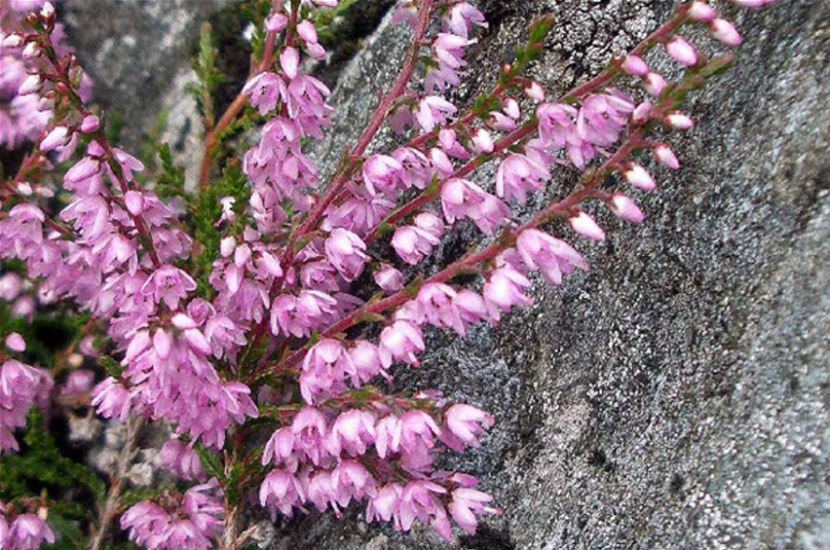

|
- |
---|---|
Background |
Perennial, hermaphrodite low shrub, widespread with a cooltemperate Eurasian oceanic distribution and wide ecological amplitude, dominant in oceanic heaths (Gimingham, 1960). Insect pollinated; spreads by outcrossed seed (Rendell & Ennos, 2002). |
Current threats |
Evidence for declines across UK and Europe due to habitat loss (afforestation, agricultural expansion and changes in pastoral management; Stevenson & Birks, 1995) and nitrogen deposition: direct effects of ammonia toxicity. Marked decreases in Calluna from increases in competitive grasses, especially in lowland heath (Bobbink et al., 2010). Upland Calluna at risk from winter damage; dwarf shrub heath at risk from heather beetle (APIS, 2019b). Grazing and burning regimes can also result in declines (Marrs et al., 2004). |
Contribution of Scottish population to total species diversity |
Scottish and Belgian populations closely related and separate from other European populations (Mahy et al., 1999a), with Scotland probably harbouring core populations. Ecotypic variation known for some flower forms and populations on toxic soil (Gimingham, 1960). |
Diversity loss: population declines |
Habitat losses due to conversion and eutrophication over centuries, have resulted in long, slow declines in heathland (Norton et al., 2009) and Calluna distribution (Braithwaite et al., 2006). Long-lived individuals and persistent seed banks (150 years) help ensure standing diversity. High levels of temporal and spatial gene flow minimize risks to genetic variation (Rendell & Ennos, 2002). |
Diversity loss: functional variation |
Negligible imminent risk of loss of adaptive / functional variation, as despite demographic changes, Calluna remains abundant and widespread in Scotland. |
Diversity loss: divergent lineages |
Negligible risk as no divergent lineages have been detected in genetic studies. |
Hybridisation/ introgression |
None known. |
Low turnover/ constraints on adaptive opportunities |
Heavy grazing can lead to loss of stands and reduction of seedling establishment (Stevenson & Birks, 1995), but seed banks have similar levels of genetic diversity as above ground plants (Mahy et al., 1999b). Overall, negligible risk from limits on turnover. |
In situ genetic threat level |
Negligible (significant population declines UK-wide, but the plant covers 16% of Scotland; no evidence of unique variation). |
Confidence in in situ threat level |
High (based on good distribution data and by direct data on most aspects of species’ biology including population level variation). |
Ex situ representation |
Accessions from two 10 km squares held at Millennium Seed Bank but no coverage of Scottish distribution. Five wild collected accessions in RBGE living collection. |
Current conservation actions |
Upland and lowland heaths are UK Biodiversity Action Plan Habitats, leading to restoration (Mitchell et al., 2008), though outcomes vary with starting conditions (Marrs et al., 2004). Attempts to curb nitrogen deposition have had some effect, but many sites exceed critical loads, and ammonia is still on the rise (Plantlife, 2017). |
Overall T13 status |
Negligible risk; Mitigation not required |
Overall T13 status explanation |
Nitrogen critical loads are still widely exceeded, but populations in Scotland are large and represent the species’ demographic stronghold. |
Assessor |
Rebecca Yahr, Royal Botanic Garden Edinburgh |
Reviewer |
Richard Ennos, University of Edinburgh |
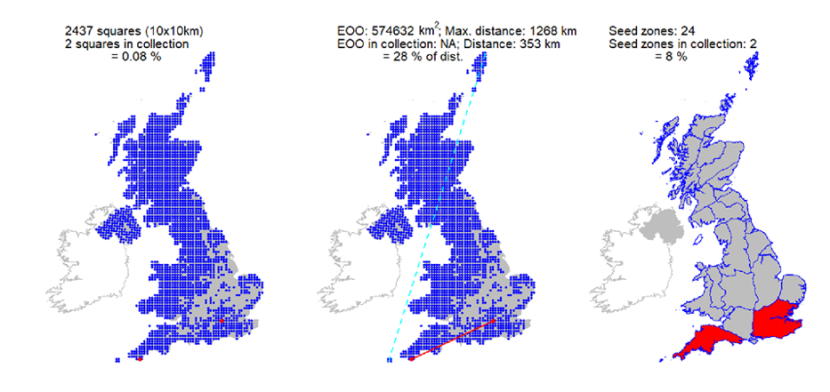
Accessions from two 10 km squares held at Millennium Seed Bank but no coverage of Scottish distribution. Five wild collected accessions in RBGE living collection.


|
- |
---|---|
Background |
Perennial, rhizomatous hermaphrodite shrub, common and locally dominant across boreal Europe and Asia, in heaths, bogs, and woodland. Pollination by insects, spreads by rhizomes; animaldispersed outcrossed seeds rarely result in seedlings in Scotland (Ritchie, 1956). Economically important wild berry. |
Current threats |
Continued gradual habitat loss of heath in lowlands and potential changes to upland vegetation from increased nitrogen deposition (APIS, 2019b; Braithwaite et al., 2006; Norton et al., 2009). Declines reported by BSBI (Braithwaite et al. 2006) and Countryside Survey (Norton et al., 2009) up to mid-2000s, probably representing population losses at range edges and loss of habitat. The species is highly susceptible to the Phytophthora pathogens P. ramorum and P. kernoviae. 22 cases have been reported in England (Fera, 2012) and two cases affecting V. myrtillus have been found in Scotland (Scottish Government Status Report, 2015) |
Contribution of Scottish population to total species diversity |
Several close relatives and subspecies are found in Europe and North America (Ritchie, 1956), all with similar life-history and regionally-distinct gene pools (Bjedov et al., 2015), but no data from UK/Scotland. Geographic and ecotypic variation in morphology and in common garden experiments (Ritchie, 1956) suggest locally adapted gene pools may be present. |
Diversity loss: population declines |
Low risk, as population declines are still small compared with the overall abundance of the species, suggesting any imminent losses of genetic diversity will be negligible. |
Diversity loss: functional variation
|
Range edge losses often correlate with losses of genetically differentiated populations (Eckert et al., 2008), but no evidence of losses in functional variation, and high-elevation populations are within stable core of distribution. |
Diversity loss: divergent lineages |
Negligible risk. No evidence of divergent lineages being under threat. |
Hybridisation/ introgression
|
Vaccinium x intermedium is a hybrid with V. vitis-ideae, found uncommonly in UK and elsewhere, but probably limited by staggered flowering times (Ritchie, 1956; Stace et al., 2015). |
Low turnover/ constraints on adaptive opportunities |
Regeneration from seed appears limited; muirburn promotes clonal re-sprouting from rootstocks (Welch et al., 1994). |
In situ genetic threat level |
Negligible (losses are currently restricted to lowlands, with Scottish populations largely intact and no evidence of unique variation here). |
Confidence in in situ threat level |
Medium (assessment based on good distribution data supported by BSBI and CEH repeat surveys; no UK direct genetic evidence). |
Ex situ representation |
Three UK accessions, from three 10 km squares held at the Millennium Seed Bank. Minimal coverage of the Scottish distribution. Representation in Royal Botanic Garden Edinburgh living collection of three wild collected UK accessions. |
Current conservation actions |
Widespread and well-characterized threats to heath from nitrogen deposition mean that actions to limit nitrogen are ongoing. |
Overall T13 status |
Negligible risk; Mitigation not required |
Overall T13 status explanation |
Core populations in Scotland are stable. Should demographic situation change (e.g. major Phytophthora outbreak), risk should be revisited. |
Assessor |
Rebecca Yahr, Royal Botanic Garden Edinburgh |
Reviewer |
Jim McIntosh, Botanical Society of Britain & Ireland Pete Hollingsworth, Royal Botanic Garden Edinburgh |

Three UK accessions, from three 10 km squares held at the Millennium Seed Bank. Minimal coverage of the Scottish distribution. Representation in Royal Botanic Garden Edinburgh living collection of three wild collected UK accessions.
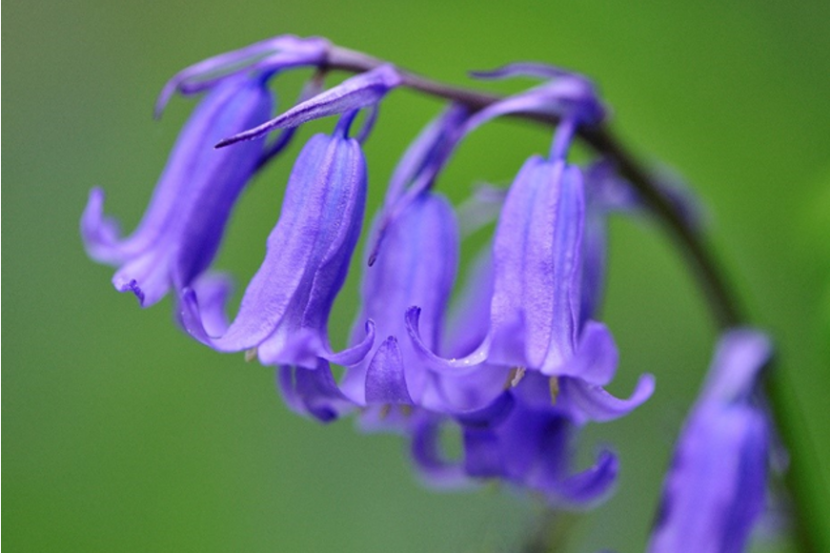
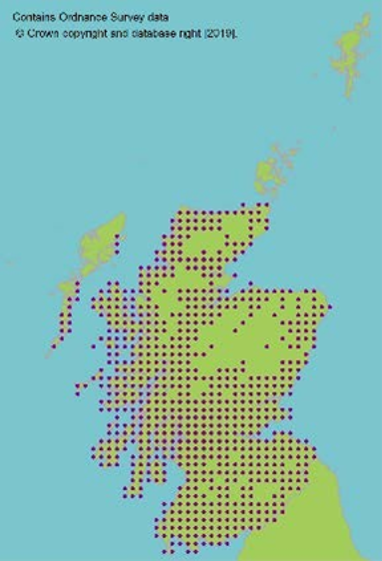
|
- |
---|---|
Background |
The British bluebell is a diploid herbaceous predominantly outcrossing perennial, able to reproduce sexually via seed and asexually via bulbs. It is widespread and abundant in the UK, with strongholds in woodlands and more open habitats towards the westcoast (Kohn et al. 2009). |
Current threats |
Introduced non-natives or “Spanish bluebells” (likely originally to have been horticultural introductions of Hyacinthoides hispanica from the Iberian peninsula and later of commercial varieties of uncertain provenance, often sold as H. hispanica but now widely ascribed to the hybrid, H. x massartiana, between H. non-scripta and H. hispanica) have been considered to be a major threat to the British bluebell either via competitive displacement or extinction by hybridisation (summarised in Kohn et al. 2019). Non-natives occur frequently throughout the range of H. non-scripta, particularly near urban areas, roadsides, and other sites of human disturbance. |
Contribution of Scottish population to total species diversity |
The British Isles are the global stronghold for the British bluebell and the species is abundant in Scotland, with many populations far from urban centres and hence likely to have more limited co-occurrence (and thus hybridisation potential) with non-natives (Kohn 2009). The Scottish population is thus globally important from a demographic perspective and may contain unique locally adapted variants. |
Diversity loss: population declines
|
No clear evidence for demographic declines. The species is widespread and abundant across the UK. There is the possibility of loss via competition with the non-native bluebell in the UK, but the current evidence of high bulb densities suggests physical encroachment is likely to have limited effects. |
Diversity loss: functional variation
|
No evidence for loss of adaptive variants. The species remains common and abundant across the UK. |
Diversity loss: divergent lineages |
As the British Isles are the global stronghold of the species, any decline in the UK runs the risk of loss of unique diversity. However, at present, there is no evidence for catastrophic loss across its range in the UK. |
Hybridisation/ introgression
|
Hybridisation has been considered a major concern. There is very high co-occurrence with non-native bluebell, and the concern is particularly acute for important large natural populations in ancient woodlands close to urban areas. However, recent genetic data shows that the native bluebell has a fertility advantage compared to non-natives, and considerably greater numbers of individuals (Kohn et al. 2009, 2019). Thus, although persistent, naturalised nonnatives are widespread and continued crossing at low levels can be expected, it is unlikely to lead to systematic and widespread genetic diversity loss in the H. non-scripta. |
Low turnover/ constraints on adaptive opportunities |
No evidence of limitation to recruitment. Populations appear to reproduce via a mixture of sexual and asexual reproduction (Blackman & Rutter 1954; Wilson 1959). |
In situ genetic threat level |
Moderate (high co-occurrence with non-native bluebells with hybridisation and competition a clear threat at individual sites; however, the reduced fertility of non-natives, perennation and reproduction by bulbs, and the numerical supremacy of the native bluebell substantially limits any threat of overall catastrophic losses). |
Confidence in in situ threat level |
High (assessment based on extensive genetic data and quantitative demographic surveys). |
Ex situ representation |
Collections from 12 10 km squares are represented in the Millennium Seed Bank, but only minimal coverage in Scotland. Two wild collected accessions represented in the living collection of the Royal Botanic Garden Edinburgh. |
Current conservation actions |
Increased awareness of the concern associated with non-native “Spanish bluebells” has led to management interventions around important sites, and discouragement of sales and planting of nonnative bluebell varieties. The British bluebell is protected under Schedule 8 of the Wildlife and Countryside Act. |
Overall T13 status |
Moderate risk; Mitigation effective |
Overall T13 status explanation |
There is a clear threat from non-native bluebells; however, the sheer numbers of the native bluebell, the longevity of individual plants via bulb production, and its greater fertility mean that in the immediate future there is limited threat to the overall genetic diversity of the British bluebell. Growing awareness of the problems from non-native bluebells should help |
Assessor |
Pete Hollingsworth, Royal Botanic Garden Edinburgh |
Reviewer |
Deborah Kohn, Royal Botanic Garden Edinburgh |
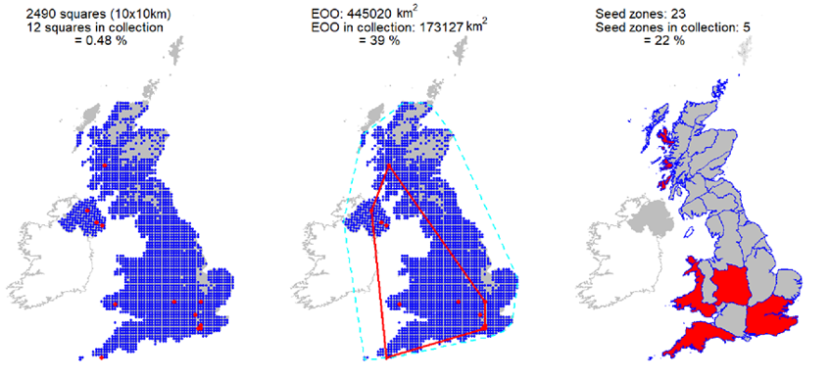
Collections from 12 10 km squares are represented in the Millennium Seed Bank, but only minimal coverage in Scotland. Two wild collected accessions represented in the living collection of the Royal Botanic Garden Edinburgh.

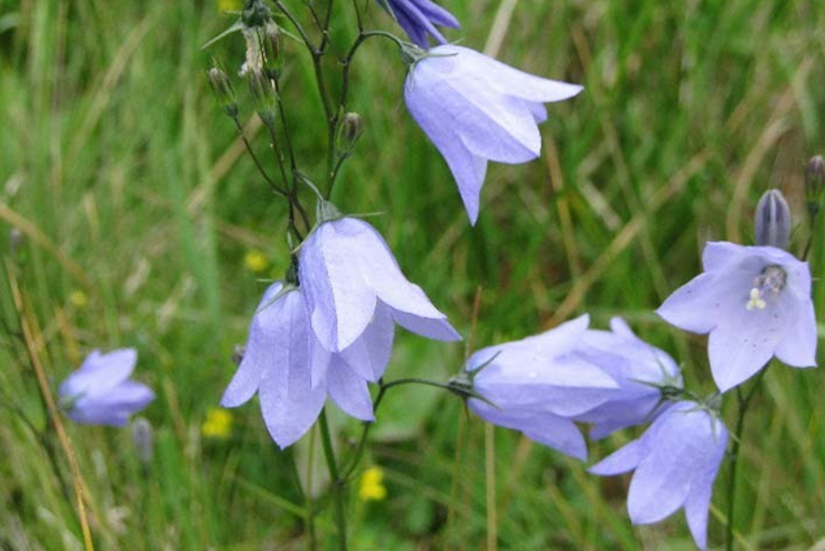

|
- |
---|---|
Background |
Perennial, hermaphrodite herb, widespread with a circumpolar distribution and wide ecological amplitude. Pollination by insects, self-incompatible, spreads by seed and rhizomes. UK populations represent two subspecies, corresponding to polyploid races (tetraploid ssp. rotundifolia and hexaploid ssp. montana) (Stevens et al., 2012). |
Current threats |
Declines in abundance and range due to intensification of agriculture and regeneration of woodlands and scrub; nitrogen deposition associated with population declines (Stevens et al., 2012). Potential for introgression from non-native genotypes planted for habitat restoration (Walker et al., 2004a,b). Seedlings are poor competitors and require disturbed open ground. Seed banks mostly persist for short intervals (<5 yrs). |
Contribution of Scottish population to total species diversity |
Hexaploid plants and susbp. montana have their strongholds in Scotland (Stevens et al., 2012). |
Diversity loss: population declines
|
Moderate risk of genetic diversity loss. Diploid race 2n=34 apparently lost from Britain (Stevens et al., 2012). Population declines reported, especially at range edges (Braithwaite et al., 2006) and 39% in Countryside Survey (as cited in Stevens et al., 2012). These population declines may lead to further genetic diversity loss. |
Diversity loss: functional variation |
Population declines have been reported in the north of Scotland (Stevens et al., 2012) and this may disproportionately affect the hexaploid cytotype / subsp. Montana. |
Diversity loss: divergent lineages |
Strong local structuring is apparent in distribution of tetraploids vs hexaploids: declines in north Scotland mostly impact the hexaploid populations (Stevens et al., 2012). |
Hybridisation/ introgression
|
Tetraploids and hexaploids are usually geographically separate, but rare hybrids occur in range overlap with occasional aneuploids. Potential for genetic problems via hybridisation with non-native genotypes in seed mixes used for habitat restoration. |
Low turnover/ constraints on adaptive opportunities |
Late summer mowing in road verges can prevent regeneration and spread from seed. |
In situ genetic threat level |
Moderate (loss of diploids from UK, losses in hexaploid ssp. montana range, continuing threat from land use change, agricultural intensification and nitrogen pollution). |
Confidence in in situ threat level |
Medium (assessment based on good distribution data supported by direct data on most aspects of species’ biology including cytotypes, but lacking a comprehensive genetic diversity study). |
Ex situ representation |
Five UK accessions, covering five 10 km squares held at the Millennium Seed Bank, but no collections from Scotland. Three wild collected accessions represented in the Royal Botanic Garden Edinburgh living collection. |
Current conservation actions |
Nitrogen deposition control beginning to show results with declines in NOx over the last decade, but approximately 40% of nitrogensensitive habitats in Scotland exceed critical loads, and ammonia still rising. Restoration projects need awareness of local provenance. |
Overall T13 status |
Moderate risk; Mitigation not in place |
Overall T13 status explanation |
A lag time between emissions controls and slowing nitrogen deposition rates is in evidence and critical loads are still being widely exceeded. Extirpation of diploid populations and declines in ssp. montana evidence loss of genetically distinctive lineages. |
Assessor |
Rebecca Yahr, Royal Botanic Garden Edinburgh |
Reviewer |
Aline Finger, Royal Botanic Garden Edinburgh Pete Hollingsworth, Royal Botanic Garden Edinburgh |

Five UK accessions, covering five 10 km squares held at the Millennium Seed Bank, but no collections from Scotland. Three wild collected accessions represented in the Royal Botanic Garden Edinburgh living collection.


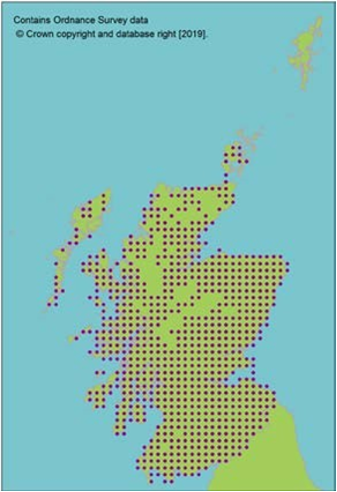
|
- |
---|---|
Background |
Subdieocious, wind-pollinated, wind-dispersed, widely distributed woodland and wayside tree. Predominantly European distribution extending into southwestern Asia. In the UK abundant locally, especially as younger trees due to recent woodland expansion (Maskell et al., 2013) and currently the second most common broadleaf tree. Soil feedbacks from high-nutrient litterfall make this a keystone species where it dominates stands. Prolific reproduction via seed and with ability to coppice, but severe risk from introduced fungal and potentially insect pests, with losses as high as 95% predicted (Thomas, 2016). Over 1000 other species are associated - with 44 restricted to ash alone in the UK – suggesting that losses of ash will result in knock-on effects for ecosystems (Mitchell et al., 2014). |
Current threats |
Severely threatened throughout the UK by the introduced ash dieback plant pathogen Hymenoscyphus pseudoalbidus (first reported in the UK in 2012). Another potential threat is from the emerald ash borer Agrilus planipennis, currently causing damage on European ash in Russia where the American green ash F. pennsylvanica has been introduced. Both of these risks can be aggravated by other environmental stressors (moisture, drought) or opportunistic pathogens (e.g. Armillaria). |
Contribution of Scottish population to total species diversity |
Scotland and the UK are part of a western European genetic lineage that colonised from an Iberian refugium. Scottish populations show local adaptation in terms of morphology and phenology, and retain high levels of genetic variation as a consequence of high pollen and seed flow, even when fragmented (Heuertz et al., 2004; Bacles et al., 2005, 2006; Whittet et al., 2019). |
Diversity loss: population declines
|
High likelihood of diversity loss. The general persistence of the species across its range in Scotland is threatened by huge predicted population losses from areas where ash dieback has been long established, with mortality ranging from ~70-85% depending on stand-type (DEFRA, 2019). Highest population losses from ash dieback are predicted for young trees, those in very moist soils and those which are ash-dominated. Presence and impacts are correlated with time since first arrival, and currently Scotland has a relatively low incidence of ash dieback. |
Diversity loss: functional variation
|
High likelihood of loss of functional variation, as locally adapted populations may encounter catastrophic losses. In all studied stands, only 1-5% of trees are suggested to be tolerant to ash dieback, but with a measurable genetic component, suggesting the potential for increasing tolerance by breeding and through natural selection in situ (Cavers & Cottrell, 2015; DEFRA, 2019). Maintaining old, healthy trees ensures production of seed and promotes continued natural selection. |
Diversity loss: divergent lineages |
Limited divergence from European populations precludes loss of major divergent lineages, but there is potential for loss of locally adapted populations. |
Hybridisation/ introgression |
No major hybridisation issue threatening genetic diversity. In some parts of Europe where ranges overlap, F. excelsior can hybridize with F. angustifolia and other species of Fraxinus. |
Low turnover/ constraints on adaptive opportunities |
High mortality and severe pressures are predicted to limit regeneration and adaptive change. Risks due to combined stresses from soil conditions, pest and pathogen pressures, and competition from faster-growing species all result in higher susceptibility to tree- and stand-scale losses, which limit healthy population turnover and adaptive evolution. |
In situ genetic threat level |
Serious (in the face of emerging pathogen threats, major population losses and limitations to regeneration present a high risk of genetic variation loss). |
Confidence in in situ threat level |
High (assessment based on good demographic data from Europe, where the effects of slow-to-manifest ash dieback can be assessed; direct data on genetic variation, population differentiation and biology). |
Ex situ representation |
Collections from 68 10km squares held at the Millennium Seed Bank, with comprehensive coverage of Scotland. It is estimated these collections capture >90% of genetic diversity (Hoban et al., 2018). They encompass 2.3 million seeds from 59 sites in 22 seed zones, representing 659 trees sampled as a case study of genetic protection (Hoban et al., 2018).
One wild collected accession is held at Royal Botanic Garden Edinburgh living collection, plus two other European wild collected and four cultivated accessions. Two UK wild collected accessions are also held in Royal Botanic Garden Kew. |
Current conservation actions |
Active projects to understand and map stresses on ash stands, understand the genetic basis for tolerance (including resistance, avoidance, the role of microbiota) and to plan breeding programmes to promote tolerance. Control of movement of nursery stocks and timber to prevent emerald ash borer. |
Overall T13 status |
Serious risk; Mitigation not effective |
Overall T13 status explanation |
Despite widespread distribution and abundance, population losses are predicted to catastrophic. Intensive management strategies, such as resistance breeding, require huge investment and benefits to wild populations and will only be realised with persistent and longterm intervention. Ex situ collections are very strong, with good representation of contemporary diversity, but do not secure a longterm future for ash genetic diversity and presence of viable and evolving ash populations. Managing populations to promote natural regeneration is the most effective way of promoting long-term adaptation to ash dieback through natural selection. |
Assessor |
Rebecca Yahr, Royal Botanic Garden Edinburgh |
Reviewer |
Richard Ennos, University of Edinburgh Peter Hollingsworth, Royal Botanic Garden Edinburgh |

Collections from 68 10km squares held at the Millennium Seed Bank, with comprehensive coverage of Scotland. It is estimated these collections capture >90% of genetic diversity (Hoban et al., 2018). They encompass 2.3 million seeds from 59 sites in 22 seed zones, representing 659 trees sampled as a case study of genetic protection (Hoban et al., 2018). One wild collected accession is held at Royal Botanic Garden Edinburgh living collection, plus two other European wild collected and four cultivated accessions. Two UK wild collected accessions are also held in Royal Botanic Garden Kew.


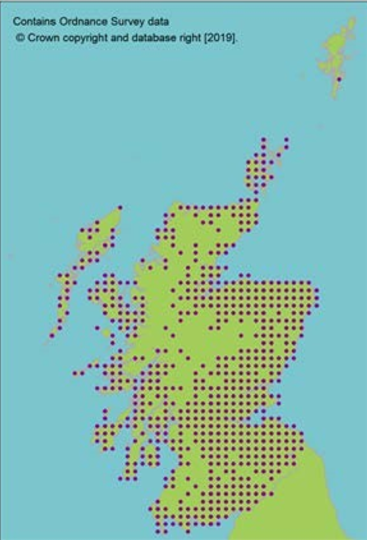
|
- |
---|---|
Background |
Perennial, hermaphrodite, deciduous shrub, common and widely distributed in eutrophic woodlands, hedges and disturbed sites in the UK and continental Europe. Pollination by generalist beetles, flies and bees. Fertilisation mostly from the same individual, though both outcrossing and fruit set without pollination have been observed. Seeds are bird dispersed (Atkinson & Atkinson, 2002). |
Current threats |
None known. This species probably benefits from periodic human disturbance and an agricultural landscape. Some sensitivity to acidification of soils for seed germination and risks to vegetative parts from late frosts and drought (Atkinson & Atkinson, 2002). |
Contribution of Scottish population to total species diversity |
The species is common elsewhere in its range and there is no expectation of divergent endemic genetic lineages in Scotland, although the populations may be adapted to the local environment. |
Diversity loss: population declines |
No evidence for genetic diversity loss and individual plant longevity ameliorates short-term risks. |
Diversity loss: functional variation
|
Populations are large and widespread and without evidence for significant decline (Braithwaite et al., 2006). No immediate risk of loss to functional variation. |
Diversity loss: divergent lineages |
Limited risk as no evidence for phylogenetically divergent lineages present in Scotland. |
Hybridisation/ introgression |
Hybrids are not reported from Britain and very rare elsewhere. |
Low turnover/ constraints on adaptive opportunities |
No constraints apparent. |
In situ genetic threat level |
Negligible (large, widespread populations and no evident threats). |
Confidence in in situ threat level |
Medium (assessment based on good demographic data supported by direct data on most aspects of species’ biology and no obvious threats; no direct genetic data available). |
Ex situ representation |
Clonally propagated collections held in 15 International Phenological Gardens in addition to accessions, covering 28 10km squares held at the Millennium Seed Bank. Comprehensive coverage of Scottish seed zones. Representation in the Royal Botanic Garden Edinburgh living collection of one wild collected accession. |
Current conservation actions |
None required. |
Overall T13 status |
Negligible risk; Mitigation not required |
Overall T13 status explanation |
With large populations, wide distribution and populations generally promoted by current landscape structure, no risks to genetic variation require attention. |
Assessor |
Rebecca Yahr, Royal Botanic Garden Edinburgh |
Reviewer |
Iain Macdonald, Scottish Natural Heritage Pete Hollingsworth, Royal Botanic Garden Edinburgh |
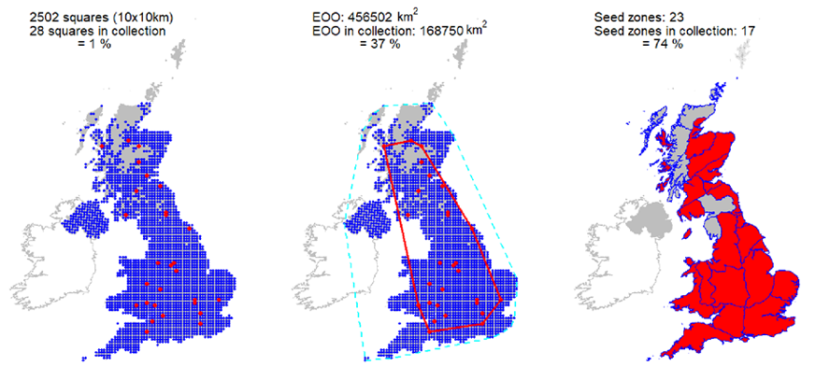
Clonally propagated collections held in 15 International Phenological Gardens in addition to accessions, covering 28 10km squares held at the Millennium Seed Bank. Comprehensive coverage of Scottish seed zones. Representation in the Royal Botanic Garden Edinburgh living collection of one wild collected accession.
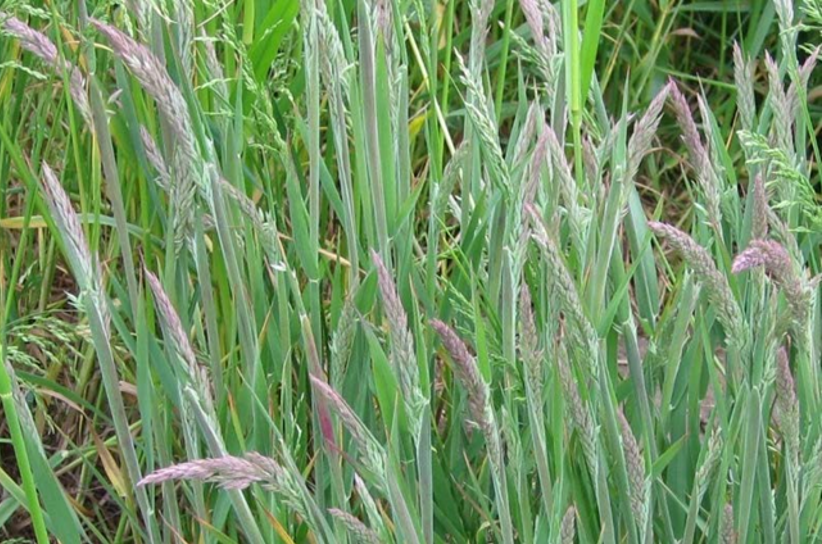
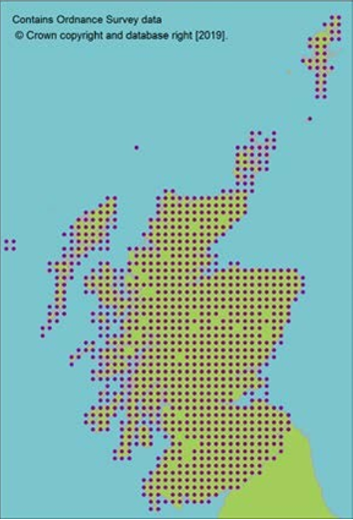
|
- |
---|---|
Background |
Perennial tufted grass, mostly outcrossing with limited potential for vegetative spread (Bischoff et al., 2006) and persistent seed banks (Beddows, 1961). One of the commonest plants in the UK (Braithwaite et al., 2006). Used in seed mixtures for restoration and revegetation and for improving grazing in native swards (Beddows, 1961; Bischoff et al., 2006). Holcus lanatus comprises two varieties, both found throughout their ranges (Rich & Jermy, 1998). |
Current threats |
Genetic swamping from introduction of non-native or non-local seed sources in restoration projects (Walker et al., 2004a). |
Contribution of Scottish population to total species diversity |
Scottish populations are likely to be among the most oceanic and may contain locally adapted genotypes. |
Diversity loss: population declines |
No evidence of declines (Braithwaite et al., 2006), but rather of human-mediated propagation for restoration. |
Diversity loss: functional variation
|
Functional variation is well characterized, but not apparently under threat: Evidence of local adaptation in seed collected from chalk vs mesotrophic grasslands (Bischoff et al., 2006); used as a model system for genetics of metal tolerance (e.g. Meharg et al., 1993). |
Diversity loss: divergent lineages |
No direct evidence of losses; widespread evidence of local adaptation to extreme conditions, e.g. metalliferous soil and climate (Macel et al., 2007). |
Hybridisation/ introgression
|
Holcus mollis is the tetraploid counterpart to diploid H. lanatus (Rich & Jermy, 1998). Some hybrids are known in the wild and can be produced by crossing (Beddows, 1961). Primary concern is hybridisation with non-local seed from restoration projects, but the species’ abundance limits the overall potential for negative impacts. |
Low turnover/ constraints on adaptive opportunities |
Large populations, reproduction mostly by outcrossed seed and ability to re-establish on disturbed sites (e.g. Lowday et al., 1992) suggests no limits to adaptive opportunities. Studies suggest a loss of biomass production due to drought (Beierkuhnlein et al., 2011). |
In situ genetic threat level |
Negligible (widespread and large populations reduce impacts of any existing pressures). |
Confidence in in situ threat level |
Medium (assessment based on good demographic data supported by direct data on most aspects of species’ biology, cytotype distribution, and no obvious threats; however, no direct genetic data available). |
Ex situ representation |
Four UK accessions, covering three 10 km squares held at the Millennium Seed Bank, but no coverage in Scotland. Representation in the Royal Botanic Garden Edinburgh living collection of one wild collected accession. |
Current conservation actions |
Recent consideration of policy shifts towards using local seed in restoration should help mitigate any potential risks from genetic swamping (De Vitis et al., 2017; Abbandonato et al., 2018) |
Overall T13 status |
Negligible risk; Mitigation effective |
Overall T13 status explanation |
Though some potential risk from genetic swamping could be pinpointed in restoration projects using commercial seed, this species is able to colonize easily without seed additions (e.g. Lowday et al., 1992). |
Assessor |
Rebecca Yahr, Royal Botanic Garden Edinburgh |
Reviewer |
Aline Finger, Royal Botanic Garden Edinburgh Pete Hollingsworth, Royal Botanic Garden Edinburgh |



|
- |
---|---|
Background |
Perennial grass forming tussocks or extensive swards, capable of spreading clonally and dominating stands. A peat-forming member of acid-grasslands, wet heath, and bog; losses in the former are contrasted with a need for controlling populations in the latter habitats. Two subspecies (tetraploid ssp. caerulea and diploid and decaploid ssp. arundinacea) are known in Britain (Taylor et al., 2001). Ecotypic variation within subspecies can be due to both genetic and ecological factors. Common and locally abundant in north and west Britain and widespread in Europe and Asia. Can form a persistent seed bank (Taylor et al., 2001). |
Current threats |
Not considered to be under threat. |
Contribution of Scottish population to total species diversity |
Locally adapted gene pools may be present, but the contribution to global species diversity is not considered particularly high. |
Diversity loss: population declines |
The distribution is broadly stable with local increases in abundance potentially due to increased nitrogen deposition, reduced grazing and / or muirburn. |
Diversity loss: functional variation |
Ecotypic variation is likely to be preserved by large populations across a wide distribution. |
Diversity loss: divergent lineages |
No evidence for divergence from European populations, precluding loss of major divergent lineages. |
Hybridisation/ introgression |
No threats known. |
Low turnover/ constraints on adaptive opportunities |
None known. Seed viability is low, but bare ground is readily colonized. |
In situ genetic threat level |
Negligible (this species is stable or locally increasing). |
Confidence in in situ threat level |
Medium (assessment based on good distribution data supported by experimental evidence on competitive abilities and quantitative changes in cover in wet heath and blanket bog habitats; no direct genetic data available for populations in Scotland). |
Ex situ representation |
Four UK accessions from three 10 km squares held at the Millennium Seed Bank, but no coverage in Scotland. |
Current conservation actions |
Burning, grazing and nitrogen regimes largely control the shifts between wet Calluna-dominated heath and Molinia-dominated heath over short and long time spans (APIS, 2019b). Recent shifts are mostly toward Molinia, especially in continental lowland heath, and attributed to increases in nitrogen. Most management interventions aim to reduce Monilia in favour of promoting Calluna. |
Overall T13 status |
Negligible risk; Mitigation not required |
Overall T13 status explanation |
Losses to genetic variation in lowlands are possible, but overall population size is very large and increasing across its range. |
Assessor |
Rebecca Yahr, Royal Botanic Garden Edinburgh |
Reviewer |
Iain Macdonald, Scottish Natural Heritage Pete Hollingsworth, Royal Botanic Garden Edinburgh |

Four UK accessions from three 10 km squares held at the Millennium Seed Bank, but no coverage in Scotland.
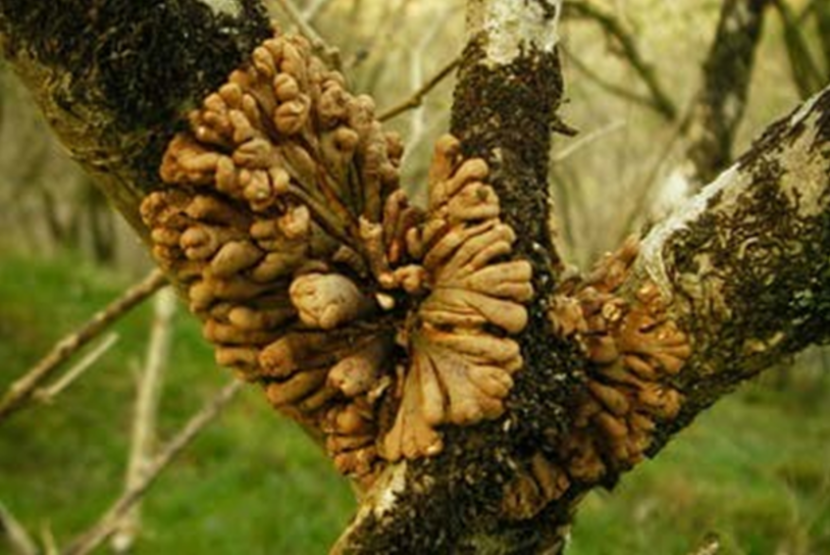
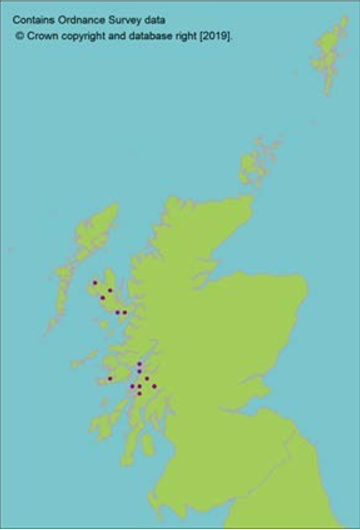
|
- |
---|---|
Background |
Hazel gloves is an ascomycete fungus parasitic on the wood-decay 'glue fungus' Pseudochaete corrugata (Grundy et al., 2012). Within Europe it is restricted to Atlantic hazel woodland (Dahlberg et al., 2010), and in North America to understory shrubs in the Appalachian woodlands (Grundy, 2014). It is a flagship species for Atlantic woodland (Coppins & Coppins, 2010). |
Current threats |
The main threats it faces in Scotland and elsewhere are fragmentation and loss of habitat; for example as a result of scrub clearance (Dahlberg et al., 2010). These issues have been exacerbated by the negative impacts of grazing and a lack of resources to ameliorate poor management, and the incursion of invasive species such as Rhododendron ponticum. |
Contribution of Scottish population to total species diversity |
Scottish and European populations show evidence of a recent population bottleneck, with source populations and higher genetic diversity in North America (Grundy, 2014). |
Diversity loss: population declines
|
Heavy grazing, concentrated grazing, coppicing and historic scrub clearance and invasive species has led to a reduction in the oldgrowth hazel habitat suitable for this species (Genney et al., 2016). However, the species is considered to be well dispersed which should retard loss of genetic diversity due to habitat fragmentation. In addition, European populations overall show very low levels of genetic variation compared to North American populations – essentially meaning that there is limited genetic variation in the first place to be lost in Scotland. |
Diversity loss: functional variation
|
Considered negligible risk as the habitat for this species is relatively homogenous, and there are no identified concerns about populations in atypical habitat that would be candidates for housing important adaptive genetic variation. |
Diversity loss: divergent lineages |
Considered negligible risk as the existing data suggest that Scottish populations contain a subset of genetic variation found elsewhere. |
Hybridisation/ introgression |
No evidence of risk of hybridisation/introgression (Grundy, 2014). |
Low turnover/ constraints on adaptive opportunities |
There is relatively high population turnover, with reproductive stromata living only for about two years. These rely on abundant populations of their host fungus (Grundy, 2014). |
In situ genetic threat level |
Negligible (relatively uncommon but well-dispersed species benefiting from favourable management which should limit future declines). |
Confidence in in situ threat level |
High (assessment based on recent genetic and ecological research). |
Ex situ representation |
Attempts to establish the species ex situ have been unsuccessful. |
Current conservation actions |
Interventions to favour the species as part of the Species Action Plan (2007-2012) led to increased awareness of the species and appropriate management of its habitat (Genney et al., 2016). Since 2017, the Atlantic Woodland Alliance has developed an action plan to meet the needs of this and other species including control of invasive species. |
Overall T13 status |
Negligible risk; Mitigation effective. |
Overall T13 status explanation |
The overall low levels of genetic diversity in the species may constrain evolutionary potential, but there is no explicit evidence of genetic problems for the species to-date. |
Assessor |
David Genney, Scottish Natural Heritage |
Reviewer |
Pete Hollingsworth, Royal Botanic Garden Edinburgh |
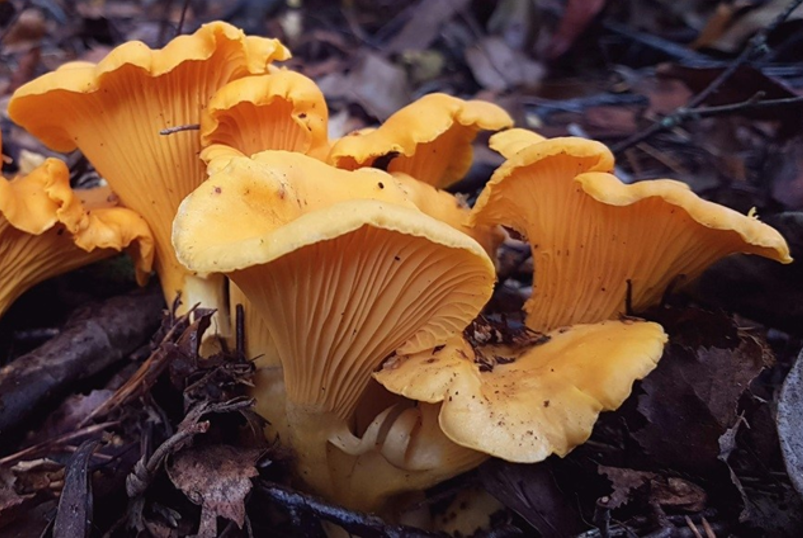

|
- |
---|---|
Background |
A locally common and widely distributed native ectomycorrhizal basidiomycete. Associated with woodland containing Betula, Fagus and Quercus species, occasionally reported in conifer plantations. Dispersal occurs via spores and vegetative growth. It is one of eight European species of Cantharellus recorded in Scotland, and it is currently considered genetically distinct from similar species in North America. It is highly prized as an edible mushroom. |
Current threats |
Not currently considered threatened (LC) although negatively impacted by loss of habitat and nitrogen deposition in parts of Europe (Pilz et al., 2003). There is a perceived localised threat from trampling resulting in soil compaction and over-picking; however, the impact is not substantiated and would not be significant at a Scottish scale. |
Contribution of Scottish population to total species diversity |
Research over the past decade has demonstrated that this is a European species distinct from North American species that were previously classified as C. cibarius (Buyck et al., 2016), and sequence diversity in public databases suggests divergent lineages even within Europe. However, there is no evidence that Scottish populations are distinct from those elsewhere in Europe. |
Diversity loss: population declines
|
No evidence for recent declines, and losses are likely to have been offset to some extent due to the species’ ability to colonise the roots of non-native species to Scotland such as beech and, possibly, commercial conifers. |
Diversity loss: functional variation |
There is no information on functional diversity within Scotland but because C. cibarius is widely distributed across Scotland, with a large population, loss of functional diversity is unlikely at present. |
Diversity loss: divergent lineages |
No clear information available, but no evidence for divergent lineages being present. |
Hybridisation/ introgression |
There is no evidence of hybridisation or introgression. |
Low turnover/ constraints on adaptive opportunities |
Turnover is a difficult concept to define for a species with an indeterminate subterranean growth form, and there is little further evidence to inform this assessment. Sexual reproduction appears to occur on a regular basis with the faithful annual production of basidiomes/fruitbodies at well-known locations. It has been suggested that intensive harvesting of the fruitbodies may cause localised suppression of long-distance dispersal, but this is not substantiated by evidence. |
In situ genetic threat level |
Negligible (the wide distribution of the fungus across a number of woodland habitats with no perceived evidence of ongoing decline leads to this assessment). |
Confidence in in situ threat level |
Medium (the lack of understanding of trend data in Scotland is likely to be outweighed by its wide distribution and likely trajectory given native woodland expansion targets). |
Ex situ representation |
The fungus can be cultured, with difficulty, on agar. It is likely that isolates are maintained in a number of mycology labs around the world but we have no evidence to substantiate this. Re-synthesis of mycorrhizal plants is fraught with difficulty and is not currently practical for reintroduction into the wild. |
Current conservation actions |
Scottish targets to increase woodland extent are likely to benefit this species. |
Overall T13 status |
Negligible risk; Mitigation not required. |
Overall T13 status explanation |
This fungus is widespread, with large populations in Scotland. No evidence for genetic distinctiveness and no evidence of population declines. |
Assessor |
David Genney, Scottish Natural Heritage |
Reviewer |
Rebecca Yahr, Royal Botanic Garden Edinburgh Pete Hollingsworth, Royal Botanic Garden Edinburgh |
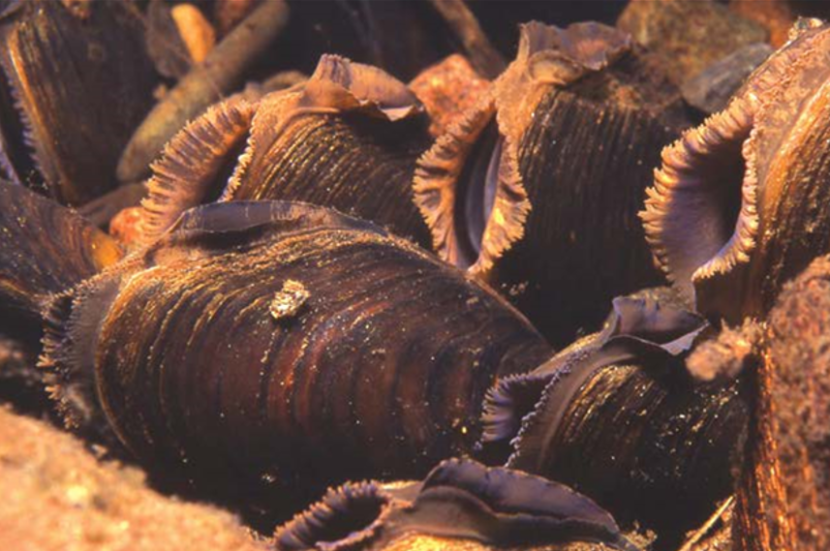

|
- |
---|---|
Background |
The freshwater pearl mussel is an endangered long-lived, bivalve mollusc present in at least 116 watercourses in Scotland, with evidence of recruitment in 74 (Cosgrove et al., 2016; Jones, unpublished data). It is one of the longest-living invertebrates, with individuals able to live for more than a century in Scotland. Historically widespread in the UK but the majority of current UK populations are restricted to the Highlands (Watt et al., 2015). The species has a long and complex lifecycle, requiring a fish host, and the juvenile stage is extremely vulnerable to habitat disturbance and damage. |
Current threats |
Pollution and siltation from surrounding land use, and the physical alteration of rivers pose the most significant threats. Illegal harvesting, the status of their host fish populations (pearl mussels reproduce parasitically on salmonid fish gills), flow modification and climate change all represent other threats (Watt et al., 2015). |
Contribution of Scottish population to total species diversity |
Scotland holds all but one of the remaining breeding populations in the UK. It also holds several of the largest remaining recruiting populations in the world. Scottish populations appear to retain a higher level of genetic diversity than populations elsewhere in Great Britain (Cauwelier et al., 2009). |
Diversity loss: population declines
|
High risk of loss of genetic diversity due to the consistent and significant population declines recorded globally, with populations in many European countries close to extinction (Lopes-Lima et al., 2017). In Scotland, freshwater pearl mussels appear to have become extinct in 73 rivers, 11 of these since 1998 (Watt et al., 2015). Some small Scottish populations show signs of population bottlenecks likely resulting in loss of genetic diversity. |
Diversity loss: functional variation
|
Limited risk, as no functional differences have been identified between populations within the UK. However, there is evidence of genetic differences between rivers, which may reflect local adaptations (Cauwelier et al., 2009) that may be lost if declines and local extirpation continues. In addition, there is some risk from changes to fish stocks as some Scottish populations prefer salmon or trout host species (Baum, 2018), which may reflect local adaptation and is associated with genetically structured populations elsewhere in Europe (Karlsson et al., 2014). |
Diversity loss: divergent lineages |
Some risk as freshwater pearl mussels in Scotland (and Northern England) belong to a single lineage, which is distinct from southern populations. |
Hybridisation/ introgression
|
No risk of hybridisation/introgression with other species. Potential risk from translocations between genetically divergent/differently adapted populations within species. |
Low turnover/ constraints on adaptive opportunities |
Long generation times of at least 10-15 years and complex lifecycle coupled with low juvenile recruitment in many populations mean that adaptive constraints are a concern in many small populations. |
In situ genetic threat level |
Serious (populations continue to be lost, with poor understanding of genetic structuring between remaining populations). |
Confidence in in situ threat level |
Medium (good data on population demography based on structured surveys but limited genetic data). |
Ex situ representation |
Captive breeding populations have been established for Irish populations, but not yet for Scottish populations. |
Current conservation management interventions |
Conservation measures underway, particularly focussed on populations with statutory designations. |
Overall T13 status |
Serious risk; Mitigation not effective |
Overall T13 status explanation |
Populations continue to be lost, despite concerted efforts. Significant declines in some populations are still predicted and, with limited understanding of local genetic variation/ adaptation, additional genetic problems may arise. Reduced juvenile recruitment is also widespread, despite significant conservation measures in many catchments. |
Assessor |
Sam Jones & Jenny O’Dell, University of the Highlands and Islands |
Reviewer |
Iain Sime, Scottish Natural Heritage Pete Hollingsworth, Royal Botanic Garden Edinburgh Linda Neaves, Royal Botanic Garden Edinburgh |

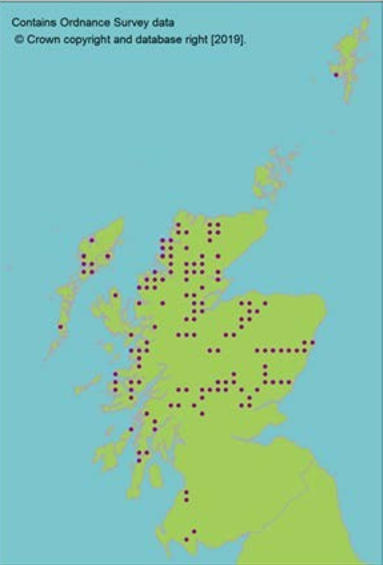
|
- |
---|---|
Background |
Widely distributed but uncommon bumblebee species, with colonies presence across Europe, Asia, and North America (Alaska). In Europe, this species has an estimated overall decline of over 30% between 2004 and 2014 (Hatfield et al., 2016) and a catastrophic range contraction in the UK (Tonhasca & Macdonald, 2016). In the UK, this species is now only found on islands off the north and west of Scotland (Inner Hebrides, Outer Hebrides, Orkney islands) and some localities in the northern Scottish mainland (Goulson, 2003). Population genetic studies showed significant genetic differentiation at large geographical scale (i.e. between the islands of South Uist, Coll, Tiree, and Orkney) but not at smaller scales (Charman et al., 2010). |
Current threats |
The main threat is land-use change resulting in loss of suitable foraging habitat to maintain the colony cycle (Jun – Sep) and sites for nesting, mating and hibernation (Charman et al., 2009). As a species with late phenology and narrow climatic niches, the increase in heat waves associated with climate change is another factor that might lead to population decline (Williams et al., 2007). |
Contribution of Scottish population to total species diversity |
Data regarding species diversity across its distribution is not available. Based on losses elsewhere in the UK, the Scottish populations will hold the last remnants of genetic diversity of the entire GB population. Distribution models predict that under climate change suitable climatic conditions in Europe will only remain in restricted areas of the Alps, Scotland and Scandinavia (Rasmont et al., 2015). Thus, Scottish populations may represent an important genetic diversity refugia in the NW of Europe. |
Diversity loss: population declines |
Substantial diversity loss has already likely occurred due to widespread declines, with lower levels of genetic diversity reported for this species in Scotland, compared to other scarce or declining bumblebees (Charman et al., 2010). Further loss is predicted if population declines/loss continue. |
Diversity loss: functional variation |
No data available on functional diversity but it is likely that adaptive variation has been lost during recent range contractions in UK given the narrowing of its extant climatic range (Rasmont et al., 2015). |
Diversity loss: divergent lineages |
No data on divergent genetic lineages within Scotland are available. |
Hybridisation/ introgression |
Low concern. No reports of hybridization or introgression for this species. |
Low turnover/ constraints on adaptive opportunities |
Low concern. Short lived species with high natural turnover. |
In situ genetic threat level |
Serious (low genetic diversity, evidence of recent major catastrophic population declines). |
Confidence in in situ threat level |
Low (demographic and genetic data give strong indication that diversity has been lost, but uncertainty as to ongoing picture). |
Ex situ representation |
No known ex-situ representation of this species. |
Current conservation actions |
Saving the Great Yellow Bumblebee conservation project has commenced, initially focusing on better understanding the species distribution, with a longer-term plan for improved habitat management. This follows on from a Species Action Framework conservation plan for this species (Tonhasca & Macdonald, 2016). |
Overall T13 status |
Serious risk; Mitigation not effective |
Overall T13 status explanation |
Major declines and now confined to a small portion of former range. Some small-scale conservation actions in place, but no certainty that the decline has been arrested, resulting in concern of ongoing loss of the remaining genetic diversity. |
Assessor |
Sílvia Pérez-Espona, University of Edinburgh |
Reviewer |
Linda Neaves, Royal Botanic Garden Edinburgh Richard Comont, Bumblebee Conservation Trust |

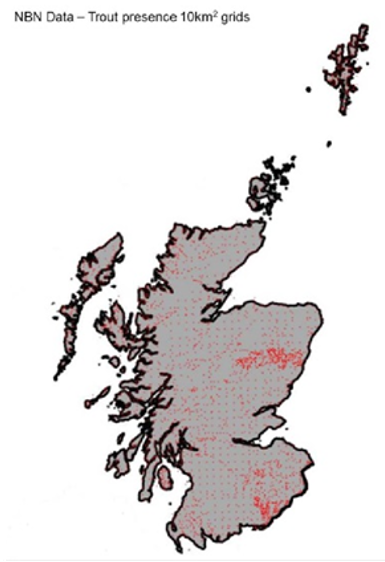
|
- |
---|---|
Background |
Native to Europe but now widely introduced across the globe (Sanz, 2018) and an important element of the Scottish angling industry. Brown trout is a polytypic species that can adopt multiple life history strategies (Klemetsen et al., 2003), which can be divided into nonanadromous (freshwater resident) and anadromous (sea-run migratory) forms. The postglacial colonization of Scotland by this species involved one or more of six lineages (see Bernatchez, 2001). Introgression may have occurred between some, but not all, lineages (Verspoor et al., 2019). Anadromous (sea) trout play a key role in maintaining gene flow between trout populations in adjacent catchments (Ferguson, 2006). Both environmental and genetic factors drive expression and maintenance of these life histories (Ferguson et al. 2019). |
Current threats |
Infections from pathogens or parasites may have contributed to local population declines of sea trout in Scotland (Urquhart et al., 2010). Higher parasitic sea louse (Leophtheirus salmonis) burdens (Moore et al., 2018) may also contribute to declines in areas near salmon farms (e.g. Thorstad et al., 2014). Hybridisation with non-native farmed stock (see Ferguson, 2007) poses a risk and has led to a restriction on the introduction of diploid fish for recreational angling, but fish farm escapes may also occur in some locations. Natural hybridisation with Atlantic salmon also occurs, although the extent and threat level are unknown (Adams et al., 2014). Environmental threats include climate change impacts, such as increased frequency of flood events or droughts, and increased river temperature (Jackson et al., 2018), while acidification, pollution and habitat fragmentation (Prodöhl et al., 2019) have had significant impacts in the past. Improvements to air and water quality, and stream connectivity may reduce the scale of these threats in future. |
Contribution of Scottish population to total species diversity |
Scottish, and particularly small, isolated populations, may have lower genetic variation than other populations in NW Europe (Vøllestad, 2018). Scotland may contain unique, potentially locally adapted genetic and phenotypic variation (Bernatchez, 2001; Duguid et al., 2006). |
Diversity loss: population declines |
Moderate risk of loss due to declines over the last decade and isolation of some brown trout populations (Palm et al., 2003). The loss of sea trout, which facilitate gene flow between adjacent catchment and support resident coastal brown trout populations, may increase the risk. |
Diversity loss: functional variation |
No data on functional diversity within Scotland, although some adaptation to local conditions may exist in isolated loch populations (Duguid et al., 2006). Irish populations show reduced Major Histocompatibility Complex diversity, possibly due to selection caused by exposure to diseases from farmed Atlantic salmon (Coughlan et al., 2006). |
Diversity loss: divergent lineages |
Limited risk as Scottish brown trout populations are part of the Atlantic lineage, which is widespread in Europe (Bernatchez, 2001). However, Scottish populations contain the highest levels of diversity within this lineage present in the UK, including some unique variants (Bernatchez, 2001; McKeown et al., 2010; Duguid et al., 2006). |
Hybridisation/ introgression
|
Hybridisation between native and non-native brown trout (or Atlantic salmon) is known to have occurred in Scotland but levels of introgression, and the impact of this, have not been assessed. |
Low turnover/ constraints on adaptive opportunities |
Data on turnover of wild brown trout populations are not available. |
In situ genetic threat level |
Moderate (relatively widespread species but multiple threats create possibility of repeated local declines/local genetic diversity loss). |
Confidence in in situ threat level |
Low (multiple threats with limited data, available genetic data is variable meaning detailed direct data required understand risks). |
Ex situ representation |
Brown trout are maintained in captive populations as part of commercial farming and stocking for angling. |
Current conservation actions |
Stocking of diploid hatchery reared fish to support recreational fisheries is being phased out (2016-2020) to avoid the potential negative impact on the genetic composition of wild populations. Where stocking is allowed, this will only be carried out using triploid stock. No conservation-focused interventions. |
Overall T13 status |
Moderate risk; Mitigation not in place |
Overall T13 status explanation |
There are multiple potential threats to local populations, and thus genetic diversity in Scotland, but relatively little baseline / monitoring data available at a national level. Conservation strategies of brown (and sea) trout populations in Scotland would benefit from large scale genetic diversity and population structure assessments, and a greater understanding of current and future risks of diversity loss. |
Assessor |
Sílvia Pérez-Espona, University of Edinburgh |
Reviewer |
Colin Bean, Scottish Natural Heritage Linda Neaves, Royal Botanic Garden Edinburgh |
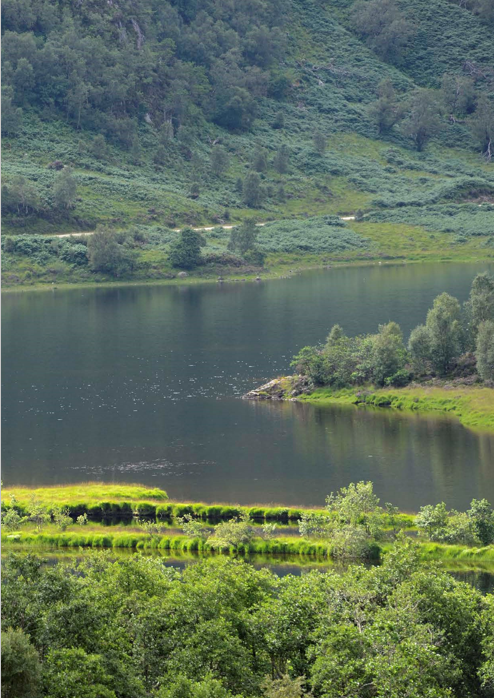

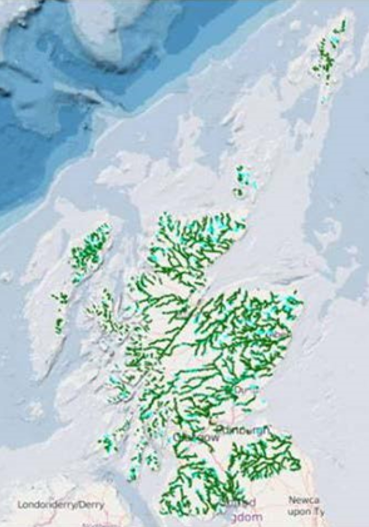
|
- |
---|---|
Background |
Globally significant species, found in the temperate and arctic regions of the northern hemisphere, and supporting commercial fishing industry and recreational angling. Present in ~389 Scottish river systems, comprising 74% of the UK stock. An anadromous species where adults return as ‘grilse’ (1-sea winter) or ‘salmon’ (multi-sea-winter) fish. Growing concerns over the decline in the numbers of grilse returning throughout Scotland and the long-term decline in the ‘Spring’ stock component due to habitat loss and other threats. |
Current threats |
Over 95% mortality in the marine environment. Climate change and by-catch of post-smolts by marine fisheries are key factors, alongside the impact of epizootic outbreaks of pathogens from aquaculture along smolt migratory routes. In freshwater, climate change, water quality and quantity, barriers to migration and invasive non-native species are also key issues. Predation also an issue. Exploitation may threaten individual populations. Stocking, to compensate for declining returns, and introgression from aquaculture escapees, may result in the loss of locally adapted traits and reduce fitness in some populations. |
Contribution of Scottish population to total species diversity |
Scottish Atlantic salmon populations make up a significant proportion of the European stock and therefore represent much of its overall genetic diversity. Analyses confirm genome-wide patterns of geographical divergence across its global (Bourret et al., 2013) and national (Gilbey et al., 2016) range. |
Diversity loss: population declines
|
High risk of loss, with catch statistics indicating a reduction in adult population size of 55% over the past 50 years. Large east coast populations appear more resilient than those in small west coast rivers and less affected by salmon farming and sea lice. Nationally, the genetically distinct ‘Spring’ MSW stock component is undergoing long-term decline. |
Diversity loss: functional variation
|
Moderate risk. Loss of genetically distinct ‘Spring’ stock present in some rivers, likely represents the loss of adaptive variation. Similarly, the occurrence of genetic differentiation across Scotland at adaptive makers, reflecting environmental variation (Cauwelier et al., 2018), suggests that populations losses may result in loss of adaptive variation. |
Diversity loss: divergent lineages |
Limited risk as Scottish populations are part of the widespread Atlantic lineage (Bourret et al., 2013), but evidence of genetic differentiation both within and between natal rivers (Cauwelier et al., 2018; Gilbey et al., 2016). |
Hybridisation/ introgression
|
Significant risk of introgression with aquaculture escapees and stocked fish, leading to loss of adaptive traits and reduced fitness in wild salmon (Gilbey et al. 2018). |
Low turnover/ constraints on adaptive opportunities |
Low adult survival and return to spawning affecting all populations linked primarily to climate change and incidental by-catch may constrain adaptive opportunities. |
In situ genetic threat level |
Moderate (relatively widespread species but multiple potential threats create the potential for genetic diversity loss).
|
Confidence in in situ threat level |
High (good demographic data and direct genetic data). |
Ex situ representation |
Farmed fish are plentiful, but do not represent wild genetic diversity; the majority having a Norwegian broodstock origin. Very limited ex situ representation of wild stocks. |
Current conservation actions |
Exploitation managed through The Conservation of Salmon (Scotland) Regulations 2016 (amended in 2019). Habitat improvements delivered through Water Framework Directive. Greater regulation of the aquaculture industry. |
Overall T13 status |
Moderate risk; Mitigation not effective |
Overall T13 status explanation |
Scottish Atlantic salmon are heavily monitored and managed but threats to genetic diversity, due to poor marine survival, sea lice, aquaculture escapees and stocking remain. Long-term future of some genetically based stock components is uncertain. |
Assessor |
Colin Bean, University of Glasgow |
Reviewer |
Martin Llewellyn, University of Glasgow Linda Neaves, Royal Botanic Garden Edinburgh |
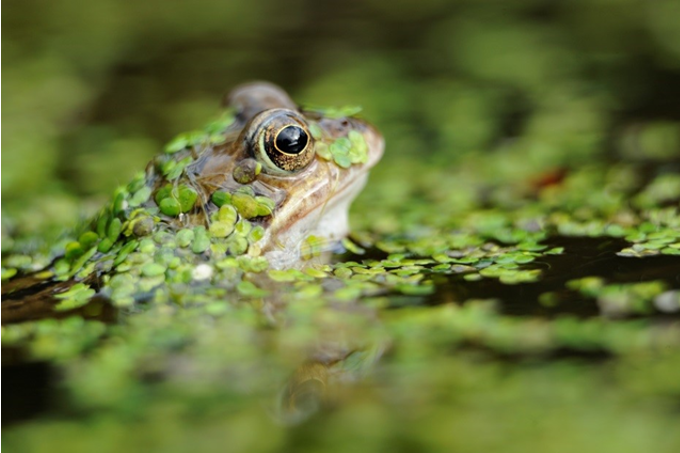
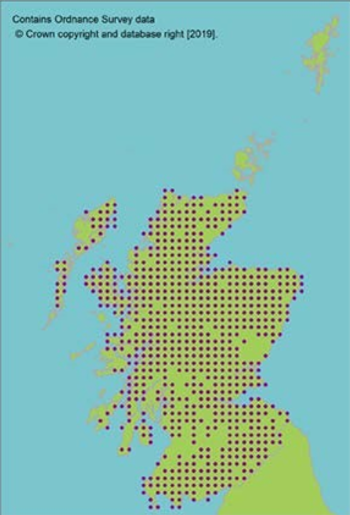
|
- |
---|---|
Background |
The common frog is a widespread species of northern and central Europe and north-western Asia. Its abundance and adaptability make it an important part of food webs both as a predator and as prey. It is found naturally throughout the Scottish mainland, including urban areas, and has been introduced to several Scottish islands (Minting, 2016). |
Current threats |
The common frog has declined across much of its European range due to loss of habitat (drainage and urban expansion), pesticide use and pollution, and novel pathogens. Although currently uncommon in Scotland, ranaviruses are predicted to spread northwards under likely climate change scenarios and may pose the greatest threat (Price et al., 2019). |
Contribution of Scottish population to total species diversity |
Mitochondrial DNA sequences from Scottish samples were identical to, or clustered with, the common haplotype previously identified from Western Europe (Muir et al., 2013). |
Diversity loss: population declines
|
Negligible risk. Although local declines have been observed, recolonisation can be rapid in urban (O’Brien, 2015) and rural areas (McKinnell et al., 2016). Frogs using urban Green Infrastructure have similar levels of genetic diversity to those in rural areas (O’Brien et al., 2017). |
Diversity loss: functional variation
|
Negligible risk of loss despite adaptations to local conditions (Muir et al., 2014), because there is high gene flow between high and low altitude populations (Muir et al., 2013) and, in urban settings, via green infrastructure (O’Brien et al., 2017). |
Diversity loss: divergent lineages |
Risk appears low as there is no evidence of structuring within Scottish populations, which themselves are little different from those of wider western European. |
Hybridisation/ introgression
|
There are no species in Scotland, native or introduced, known to produce fertile hybrids with common frog, although introductions of conspecifics from elsewhere in its range have occurred. |
Low turnover/ constraints on adaptive opportunities |
Common frogs in Scotland typically produce up to 2000 eggs per breeding pair and can use a wide range of water bodies to breed and reproduce readily (Minting, 2016). |
In situ genetic threat level |
Negligible (genetically diverse, with evidence of gene flow between populations despite natural and artificial barriers). |
Confidence in in situ threat level |
High (assessment based on good demographic data, direct data on genetic variation, population differentiation and biology). |
Current conservation actions |
The common frog benefits from the creation of generalist wildlife ponds in urban and rural areas. The Green Infrastructure Strategic Intervention is creating new habitats for urban amphibians. |
Overall T13 status |
Negligible risk; Mitigation not required
|
Overall T13 status explanation |
Despite post-war declines in both rural and urban areas, the high fecundity, population connectivity and colonisation ability of the species suggest low risk of genetic loss. However, that very connectivity leaves it at risk of novel pathogens and must inform future management. |
Assessor |
David O’Brien, Scottish Natural Heritage |
Reviewer |
Robert Jehle, University of Salford Pete Hollingsworth, Royal Botanic Garden Edinburgh Linda Neaves, Royal Botanic Garden Edinburgh |
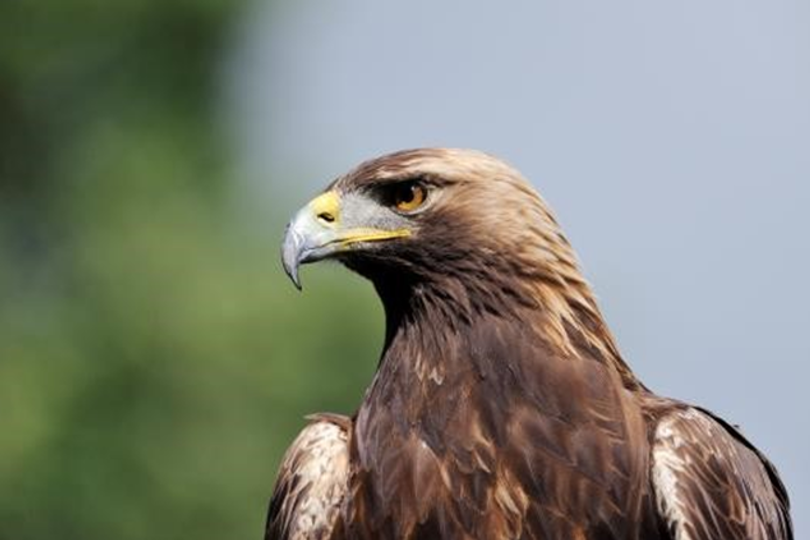

|
- |
---|---|
Background |
Iconic raptor that has suffered historic reductions in range and population size across the UK. Population currently limited to Scotland but are considered stable/recovering (Watson, 2010; Hayhow et al., 2017). |
Current threats |
Ongoing persecution, particularly in East Scotland threatens maintenance/growth of eagle populations in the region. Reproduction rates in certain other areas are considered below expectation, with unknown causes but shortage of live prey is likely a key factor and subject of current research. |
Contribution of Scottish population to total species diversity |
Scotland is the only GB region with golden eagles, however, the contribution to global species diversity is not considered particularly high. Part of a circumglobal species distribution. |
Diversity loss: population declines
|
Negligible risk as the population is considered stable and the Scottish golden eagle population is not heavily structured (Ogden et al., 2015), meaning the loss of genetic diversity through individual population declines is limited. However, low population numbers elevate sensitivity to diversity loss through random genetic drift, especially outwith the Scottish Highlands and Islands core range. |
Diversity loss: functional variation
|
Population unlikely to have strong local adaptive diversity within Scotland, minimising risk of loss to functional variation at a national scale. Functional differentiation of Scottish birds within the species unknown but subject of ongoing research. |
Diversity loss: divergent lineages |
Risk of loss considered low. One DNA lineage observed in Scotland not seen elsewhere to date, however, Scotland shares many other lineages with populations across Eurasia (Nebel et al., 2015). |
Hybridisation/ introgression |
No risk of hybridisation/introgression. |
Low turnover/ constraints on adaptive opportunities |
Very low population numbers/recruitment in certain regions (e.g., southern Scotland) and Northern Ireland may limit range of adaptive responses. |
In situ genetic threat level |
Negligible (stable and sustainable population of a vagile species with no imminent threat of diversity loss). |
Confidence in in situ threat level |
High (high-quality genetic and demographic data). |
Current conservation actions |
Close annual monitoring of breeding success through national surveys (Hayhow et al., 2017) and ringing studies. Ongoing south Scotland translocation project to reinforce population across southern counties. |
Overall T13 status |
Negligible risk; Mitigation effective |
Overall T13 status explanation |
The overall population is currently stable/increasing. Measures are in place to increase small isolated sub-populations. Lack of observed genetic structure within Scotland and sharing of evolutionary lineages beyond Scotland minimises risk of losing genetic diversity. |
Assessor |
Rob Ogden, University of Edinburgh |
Reviewer |
Des Thompson, Scottish Natural Heritage Linda Neaves, Royal Botanic Garden Edinburgh |
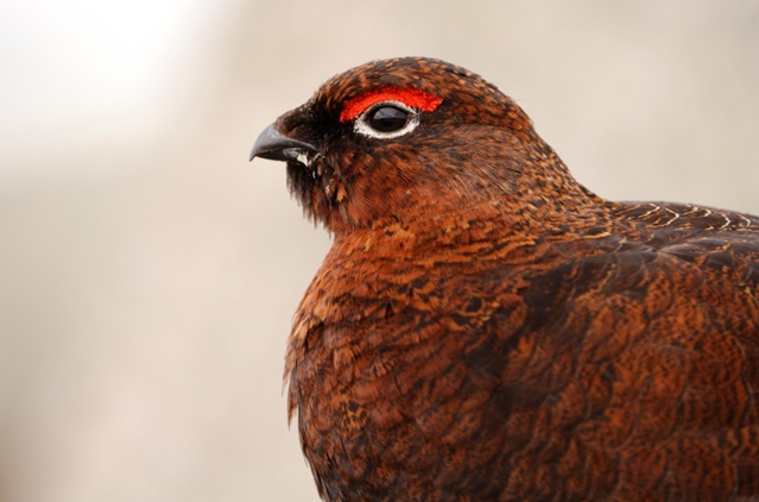
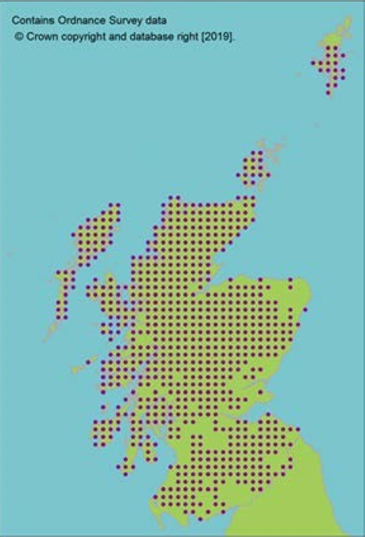
|
- |
---|---|
Background |
Iconic upland species widely distributed in heather (Calluna vulgaris) dominated moorland areas. Culturally and economically important in rural Scotland as game species, with most revenue obtained through sport hunting (Storch, 2007). Long-term declines of this species in range and abundance have been reported (Eaton et al., 2009). Declines are associated with the loss or deterioration of heather moorland habitats, as a result of land use change from management of the habitat for hunting to an increase in sheep grazing and forestry plantations (Robertson et al., 2001, 2017; Ludwig et al., 2018). There is no evidence of lower than expected levels of genetic diversity across either functionally important or neutral markers given the population size (Piertney et al., 1998; 2000; Quintela et al., 2010; Meyer-Lucht et al. 2016). |
Current threats |
The main threat to red grouse is the disappearance of heather moorland, an upland habitat with internationally recognised conservation importance (Robertson et al., 2017). Other threats include infections by the nematode Trichostrongylus tenuis and the louping ill virus, and predation by avian and mammalian species (Martínez-Padilla et al., 2014). |
Contribution of Scottish population to total species diversity |
Red grouse is considered a subspecies of willow grouse (Lagopus lagopus) and has been shown to be genetically distinct using both neutral and adaptive genetic markers (Kozma et al., 2019). Scottish grouse have also been shown to be genetically distinct from the Irish subspecies L. l. hibernicus. As an endemic subspecies of northern and western Britain, Scottish populations contribute significantly to overall species diversity. |
Diversity loss: population declines
|
Population genetic studies have found no evidence of reduced genetic diversity in red grouse, though the spatial pattern of genetic variation is affected by landscape features, social structure and levels of population isolation. Continuing habitat loss and population fragmentation could lead to diversity loss. |
Diversity loss: functional variation
|
There are no genome-wide studies of variation in genetic diversity across populations from Scotland. Genetic variation at several adaptive genetic markers (Wenzel et al., 2015 a,b,c) and patterns of epigenetic methylation (Wenzel & Piertney, 2014) have been shown to be affected by parasite burden of the gastrointestinal nematode T. tenuis. |
Diversity loss: divergent lineages |
Red grouse is a distinct genetic lineage from willow ptarmigan (L. lagopus) and the Irish subspecies (L. l. hibernicus) (Quintela et al., 2010; Höglund et al., 2013; Meyer-Lucht et al., 2016). |
Hybridisation/ introgression
|
Limited risk. Hybridisation between red grouse and willow ptarmigan can occur in captivity (Sharp & Moss, 1981), although the geographic isolation between these forms means in the wild means hybridisation does not occur in natural populations. There is some evidence of occasional introgression between red grouse and ptarmigan (L. muta) where their distributions overlap. |
Low turnover/ constraints on adaptive opportunities |
Relatively short generation time and no observed loss of reproductive fitness. |
In situ genetic threat level |
Moderate (widely distributed species but immediate threats due to land use change and disappearance of heather moorlands) |
Confidence in in situ threat level |
High (detailed data on land use change in Scotland and population declines associated with disappearance of heather moorland, direct genetic data). |
Current conservation actions |
The fate of this species is tightly linked to its management as a game species, i.e. maintenance of heather moorland and control of parasites and predators; Robertson et al. (2017). |
Overall T13 status |
Moderate risk; Mitigation effective |
Overall T13 status explanation |
Disappearance of habitat threatens population sustainability, although commercial management interventions in game regions is likely to support red grouse numbers in the immediate future. |
Assessor |
Sílvia Pérez-Espona, University of Edinburgh |
Reviewer |
Stuart Piertney, University of Aberdeen Linda Neaves, Royal Botanic Garden Edinburgh |
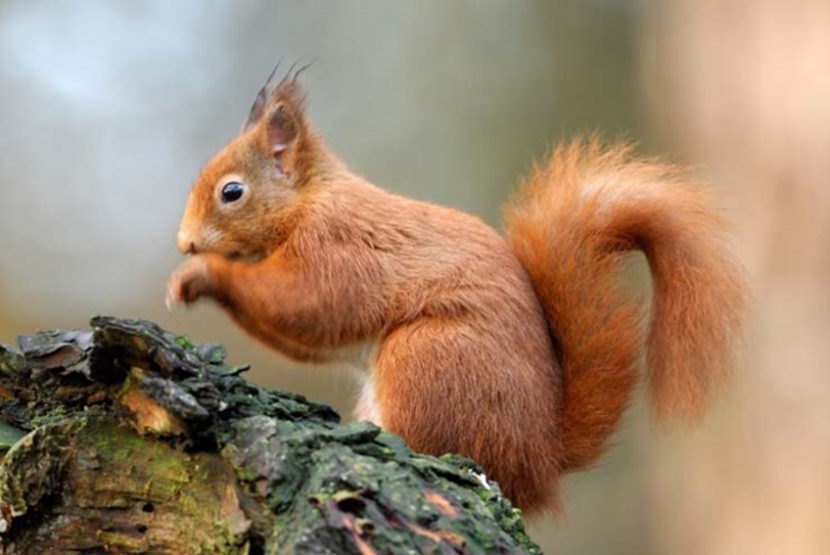
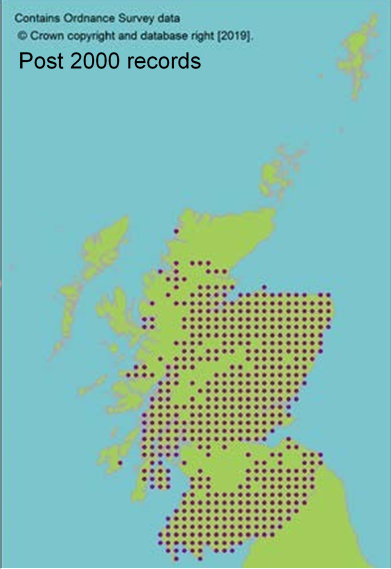
|
- |
---|---|
Background |
Iconic UK mammal species found in woodland habitats with a wide Eurasian distribution. Within the UK, there have been many translocations of red squirrels. It is a flagship species for many nature reserves. |
Current threats |
Habitat competition and displacement by invasive grey squirrel, Sciurus carolinensis (Kenward et al., 1998). Loss of reproductive fitness and risk of death due to diseases, including squirrelpox virus, adenovirus and potentially leprosy (McInnes et al., 2006; Avanzi et al., 2016). |
Contribution of Scottish population to total species diversity |
No unique lineages currently recognised in the UK (Barratt et al., 1999), despite a historical description of a UK subspecies. The Scottish populations represent the last remaining UK stronghold. Contribution to total diversity across the species range is not high but may harbour unique genotypes and adaptations due to its edge of range distribution. |
Diversity loss: population declines
|
Risk of ongoing diversity loss through population declines in southern Scotland. Lack of knowledge of population structure or distribution of genetic diversity hampers risk assessment of local extirpation on genetic diversity in Scotland. |
Diversity loss: functional variation
|
Reduced immunogenetic diversity relative to European populations observed (Ballingall et al., 2016) but potential rate of loss unknown. Scottish population is edge-of-range and may contain locallyadapted genetic variants. |
Diversity loss: divergent lineages |
Risk considered negligible, as evolutionary lineages were derived from continental Europe relatively recently. Phylogeographic studies show no distinct monophyletic lineages in England and Wales; Scotland poorly studied. |
Hybridisation/ introgression
|
No risk of hybridisation/introgression with other species. Unmanaged translocations risk undesirable introgression between divergent populations. |
Low turnover/ constraints on adaptive opportunities |
Species reproduces annually with sufficient fecundity for rapid mammalian population growth. |
In situ genetic threat level |
Moderate (clear threats from competition/disease set against extensive conservation management for red squirrel and its habitat). |
Confidence in in situ threat level |
Medium (red squirrels have been subject to detailed studies in Scotland resulting in its conservation status being well understood and the species has been subject to many genetic studies elsewhere). |
Current conservation actions |
Active conservation management of red squirrel populations in the Scottish borders, maintenance of a grey squirrel boundary line across the country, south of the Highlands. Forest and Land Scotland currently considers promotion of red squirrel habitat within strategic planning. Some ad-hoc translocation programmes underway to populate the western Highlands from central and eastern areas. |
Overall T13 status |
Moderate risk; Mitigation effective |
Overall T13 status explanation |
Populations considered stable in most of Scotland. The replacement of red squirrels by greys seen throughout England and Wales has been retarded in southern Scotland, and grey squirrel encroachment into the Highlands has currently been prevented. A lack of molecular genetic data across Scotland prevents identification of possible population genetic structure or genetic diversity in Scotland. |
Assessor |
Rob Ogden, University of Edinburgh |
Reviewer |
Peter Lurz, University of Edinburgh Linda Neaves, Royal Botanic Garden Edinburgh |
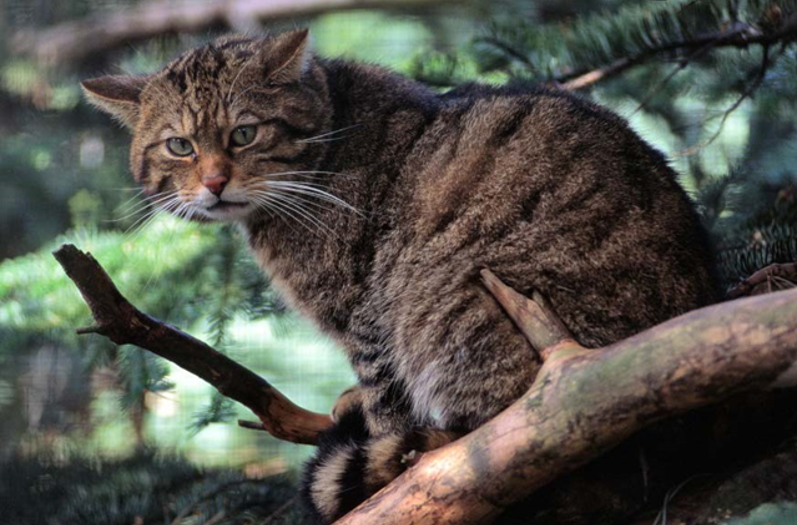
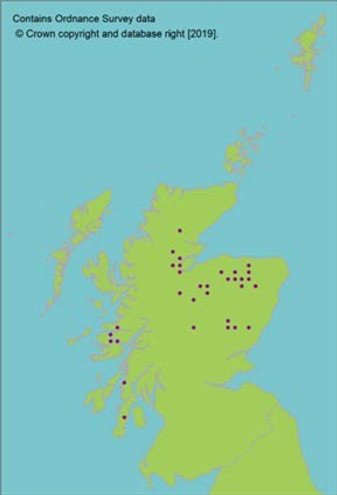
|
- |
---|---|
Background |
A Scottish icon and the only remaining native British cat. Scottish wildcats are a subpopulation of the European wildcat (Kitchener et al. 2017), although previously described as a subspecies. Although once found throughout the UK, they are now restricted to Scotland due to declines resulting from persecution and possibly other drivers, such as habitat change. The range contracted to the far north west before recovering to areas north of the Highland boundary fault (Langley & Yalden, 1977). Further contractions occurred in 21st C, due to illegal or accidental persecution and/or declines in prey (rabbit) populations, with no recent records from the north west of Scotland (Sainsbury et al., 2019). Genetic analysis has shown existence of a hybrid swarm, with all wildcats showing some hybridisation and most showing significant introgression (Senn et al., 2018). Hybridisation risks misidentification and consequently wildcats being killed during legal predator control by land managers. |
Current threats |
Primary threats are genetic introgression with domestic cat, Felis catus and persecution. |
Contribution of Scottish population to total species diversity |
The species is common elsewhere in its range and there is no evidence of divergent genetic lineages in Scotland, although the populations may be adapted to the local environment. |
Diversity loss: population declines
|
There is a high risk of diversity loss associated with recent rangecontractions and most remaining populations being small, fragmented and isolated. Low population numbers elevate sensitivity to diversity loss through random genetic drift and inbreeding, although no data is available on genetic relatedness within the remaining populations. (Introgression is the primary threat in all populations; see below). |
Diversity loss: functional variation |
No data available. Functional variation may be retained within hybridised individuals, but populations lost from the far north and west may have had unique adaptations to those environments. |
Diversity loss: divergent lineages |
Limited risk as no evidence for phylogenetically divergent lineages in Scotland. Kitchener et al. (2017) concluded there was insufficient evidence to recognise the wildcats in Scotland as a separate subspecies from F. s. silvestris in central and western Europe. |
Hybridisation/ introgression |
Serious risk. Wildcats in Scotland have very high rates of introgressive hybridisation with domestic cats. Since 2014, all wildliving cats sampled have shown domestic cat ancestry (Senn et al., 2018). Typically, phenotypic wildcats exhibit 60-80% wildcat genetic heritage. |
Low turnover/ constraints on adaptive opportunities |
Excessive influx of domestic cats into the wild-living population could limit adaptive opportunities but insufficient data is available to confidently state this. Hybrids may be better adapted to a humaninfluenced landscape than wildcats, but not enough research has been conducted to be confident of this. |
In situ genetic threat level |
Serious (small fragmented populations of hybridising species present genetic risks). |
Confidence in in situ threat level |
High (assessment based on public records across the range and intensive camera trap surveys repeated in Wildcat Priority Areas, supported by direct data on genetic introgression). |
Ex situ representation |
Genetic tests on individuals held in captivity show around 80 cats with low or very low (near zero) introgression with domestic cat. However, initial genetic analysis and studbook records suggest the captive population is inbred and new genetic variation is needed. Two wildcats taken from the wild have been included in this breeding programme but it is likely that individuals will need to be obtained from other parts of the European wildcat range. |
Current conservation actions |
Thresholds based on appearance (a pelage score) and, when available, genetic tests have been set to ensure wildcats are not accidentally neutered or killed, and that highly introgressed individuals are neutered. Conservation focused in five Wildcat Priority Areas and includes the neutering (and vaccinating) of feral cats and campaigns for neutering and vaccination of pets. Local education on the risk to wildcats from pet cats and land management activities such as predator control. |
Overall T13 status |
Serious risk; Mitigation not effective
|
Overall T13 status explanation |
Current conservation efforts will not halt cryptic extinction. As a minimum, supplementing existing populations with wildcats from captivity or from elsewhere in the wildcat’s range in Europe is needed. This has not yet begun. |
Assessor |
Roo Campbell, Scottish Natural Heritage |
Reviewer |
Rob Ogden, University of Edinburgh Linda Neaves, Royal Botanic Garden Edinburgh |
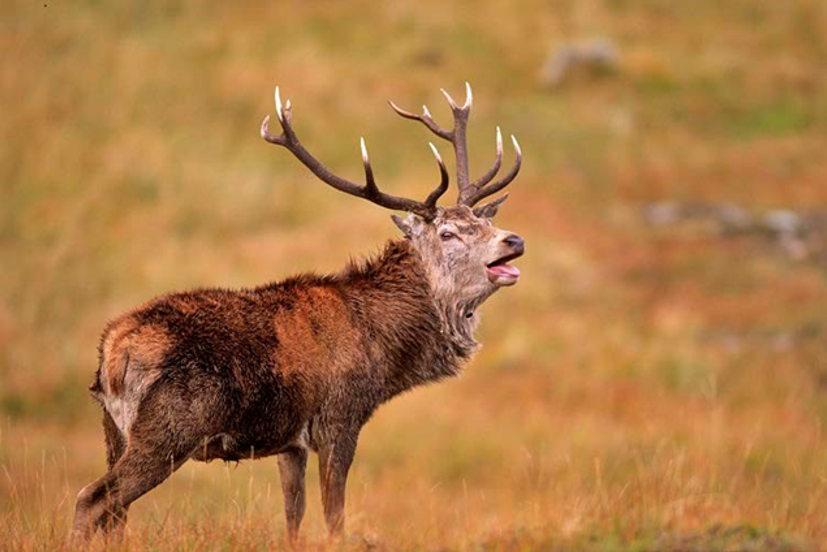

|
- |
---|---|
Background |
Ecologically important species for the maintenance of upland diversity and an important element of the Scottish rural economy through revenue from stalking, venison and tourism, as well as a valued Scottish cultural icon (Pérez-Espona et al., 2009b). The current Scottish population is c. 445,000 – 505,000, with populations widely-distributed across the mainland and most of the islands (SNH, 2016). Overall genetic diversity measured at multiple markers is considered high (Pérez-Espona et al., 2009a; 2019). |
Current threats |
The main threat is hybridization with sika deer (see Hybridisation section). The risk and manifestation of disease in European wild deer is not as high as that reported in North America but has increased in the past years, e.g., detection of Chronic Wasting Disease in Norway, introduction and spread of bluetongue virus (BTV), re-emergence of bovine tuberculosis (bTB), and prevalence of paratuberculosis in red deer (Ferroglio et al., 2011). |
Contribution of Scottish population to total species diversity |
Scotland holds the largest red deer population in Europe (Burbaite & Csányi, 2010; Pérez-Espona et al., 2009b). Genetic diversity has been found to be similar or higher to other European populations (Pérez-Espona et al., 2008; 2009a), with high genetic diversity at the Major Histocompatibility Complex DRB exon 2 loci (Pérez-Espona et al., 2019). |
Diversity loss: population declines
|
The current large populations of red deer in Scotland suggest there is no threat of diversity loss due to population declines. |
Diversity loss: functional variation
|
No evidence of risks to loss of functional variation. |
Diversity loss: divergent lineages |
Scottish populations are part of the Western European red deer lineage (Pérez-Espona et al., 2009a; Skog et al. 2009). Scottish population may harbour unique diversity but risks are negligible given the current population sizes. |
Hybridisation/ introgression |
Hybridisation between red deer and sika deer Cervus nippon is one of the main threats to the maintenance of genetic distinctiveness in Scottish red deer populations. Hybrid individuals are mainly found on the Kintyre Peninsula (Senn & Pemberton, 2009; Smith et al., 2018), with smaller numbers in the North Highlands. No hybrids have been detected in the Central Highlands or the Hebrides (Smith et al., 2018) but this is likely underestimated due to limitations of detection. |
Low turnover/ constraints on adaptive opportunities |
No indication of limited recruitment that would constrain adaptive opportunities.
|
In situ genetic threat level |
Negligible (large populations with high genetic diversity in mainland Scotland; limited hybridisation with sika deer; CWD not reported in Scotland). |
Confidence in in situ threat level |
High (direct estimates of genetic diversity and levels of hybridisation). |
Ex situ representation |
Red deer individuals with Scottish origin can be found in deer parks and in New Zealand wild populations, although it is likely that some ex situ populations are introgressed after crossing with other red deer populations and North American wapiti (Cervus canadensis). |
Current conservation actions |
Deer populations managed for production and to control densities in certain habitats; not directly managed for species conservation. |
Overall T13 status |
Negligible risk; Mitigation not required
|
Overall T13 status explanation |
Population size in Scotland is large and stable. Good information on genetic diversity across Scotland, although further areas need to be studied for a complete overview. In particular, genetic studies need to be conducted in Dumfries and Galloway (southwest Scotland); areas were sika deer have expanded. |
Assessor |
Sílvia Pérez-Espona, University of Edinburgh |
Reviewer |
Josephine Pemberton, University of Edinburgh Linda Neaves, Royal Botanic Garden Edinburgh |
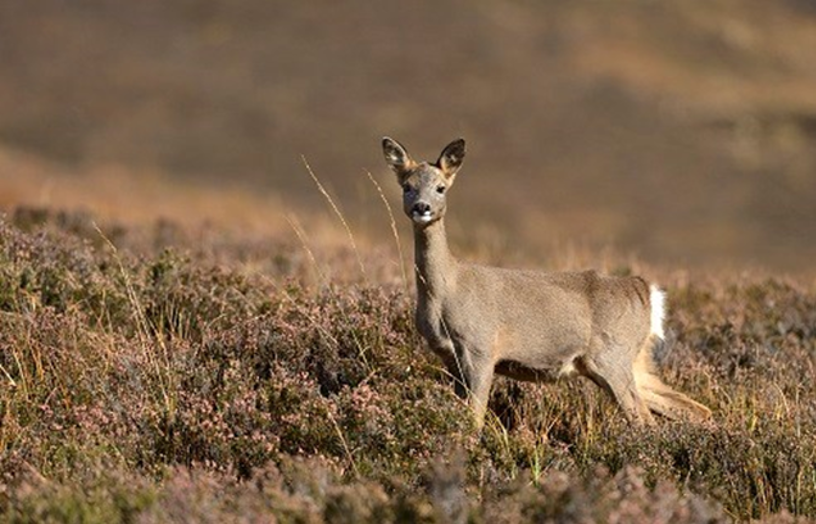
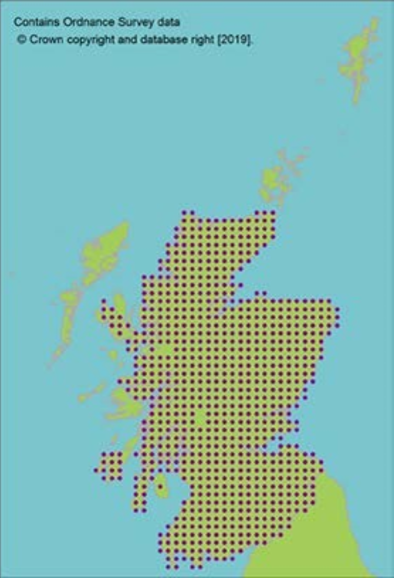
|
- |
---|---|
Background |
Iconic forest-dwelling species. Economically important game species, due to the revenue gained through deer stalking, venison production, and tourism. Although associated with woodland, it is increasingly found in urban areas due to its ecological plasticity and adaptability. Numbers were drastically reduced by overhunting and deforestation but remnant populations are thought to have remained in some areas in Scotland (Whitehead, 1964). Marked expansion reported since 1972 with roe deer now found across most of the Scottish mainland and some islands (Ward, 2005). Total current estimated population size of 200,000 – 350,000, with the highest densities found in lowland areas (Edwards & Kenyon, 2013). |
Current threats |
The main threat to populations in Scotland is introgression from nonnative stock (see Hybridisation section). Other potential threats to populations may arise as the result of emergence and re-emergence of disease, or due to transmission of disease from livestock. Disease manifestation and risk in European wild ungulates is not as high as that reported in North America, but has increased in the past years, e.g. detection of Chronic Wasting Disease in Norway, introduction and spread of BTV, re-emergence of bTB, and prevalence of paratuberculosis (Ferroglio et al., 2011). |
Contribution of Scottish population to total species diversity |
Despite an increase in harvest of 735% over the last twenty years, the UK holds one of the largest populations in Europe, with the largest continuous population being found in Scotland (Burbaité & Csányi, 2009). There is a lack of studies assessing genetic diversity of roe deer across Scotland, relative to the species range. |
Diversity loss: population declines |
Considering the sizeable range expansion and large populations in Scotland, there are no apparent current threats that would lead to population declines and genetic diversity loss.
|
Diversity loss: functional variation
|
There are no studies regarding functional variation in Scottish populations. |
Diversity loss: divergent lineages |
No evidence for divergent lineages but Scottish populations contain unique diversity not found elsewhere in the UK (Baker & Hoelzel, 2013). Risk of loss is negligible given current population trends. |
Hybridisation/ introgression |
Hybridisation with other species is unlikely, as no introductions of Siberian roe deer have been reported. Introductions of a small number of non-native roe deer stock in Strathclyde and Dumfries during the 18th and 19th centuries have been documented (Baker & Hoelzel, 2013) but detailed genetic studies in these areas are not available. |
Low turnover/ constraints on adaptive opportunities |
No indication of limited recruitment that would constrain adaptive opportunities. Roe deer is the most widely distributed species of deer in Scotland, occupying most of the mainland. Densities vary in forested areas but are particularly high in lowland areas. The species’ rapid increase reported from the 1970s onward suggests recruitment is not limiting. |
In situ genetic threat level |
Negligible (large expanding populations in Scotland presenting adaptability to a range of habitats; introduction of non-native stock not a current management practice; no imminent threats identified). |
Confidence in in situ threat level |
High (good demographic data on range expansion; some direct genetic data). |
Ex situ representation |
Scottish roe deer genetic diversity is likely to be represented in English roe deer populations as a result of re-introductions (Whitehead, 1964). Roe deer from Scottish populations may be represented in deer parks. |
Current conservations actions |
None |
Overall T13 status |
Negligible risk; Mitigation not required
|
Overall T13 status explanation |
Population size in Scotland is large and stable. No hybridisation risk. Some existing data on genetic diversity relative to UK and continental European populations. Further studies on the impact of past reintroductions in the genetic make-up of Scottish populations would be particularly useful. |
Assessor |
Sílvia Pérez-Espona, University of Edinburgh |
Reviewer |
Josephine Pemberton, University of Edinburgh Linda Neaves, Royal Botanic Garden Edinburgh |
Technical note on interpretation of maps of ex situ representation in seed banks
For seed plants (angiosperms and conifers), where major structured seed banking programmes are underway, we present maps at the 10 km scale summarising the degree to which the seed collections represent the species distribution. The baseline data for these maps are from the Botanical Society of the British Isles.
The maps are structured as follows:

The maps are structured as follows:
- The left side map shows the distribution of the species in 10 km squares in the UK, with blue squares indicating presence and red squares showing where the species occurs and which are represented in the Millennium Seed Bank (MSB).
- The middle map shows the Extent-of Occurrence (EOO) of the species in the UK (pale blue convex polygon around all 10 km square records), and the EOO of the sampled squares represented in ex situ collections in the MSB (in red). For situations where three or more 10 km squares are represented in the MSB, the proportion of the range that is represented by the ex situ collection is summarised. When only two squares are represented, area calculations are not relevant and we report the linear distance between these 10 km squares as a proportion of the maximum linear distance between occupied 10 km squares. Where only a single square is represented in the MSB, this metric is undefined.
- The right side map shows the number of seed zones in Great Britain where the species occurs, with the red shading indicating the number of seed zones that are represented in the MSB.
References
Abbandonato, H., Pedrini, S., Pritchard, H.W., De Vitis, M. & Bonomi, C. 2018. Native seed trade of herbaceous species for restoration: a European policy perspective with global implications. Restoration Ecology, 26, 820-826.
Adams, C.E., Burrows, A., Thompson, C. & Verspoor, E. 2014. An unusually high frequency of Atlantic salmon x brown trout hybrids in the Loch Lomond catchment, west-central Scotland. The Glasgow Naturalist, 26, 75-81.
APIS, 2019b. Nitrogen deposition - dwarf shrub heath.
Atherton, I., Bosquanet, S., & Lawley, M. eds. 2010. Mosses and Liverworts of Britain and Ireland: a field guide. British Bryological Society, Plymouth.
Atkinson, M.D. & Atkinson, E. 2002. Sambucus nigra L. Journal of Ecology, 90, 895-923.
Avanzi, C., Del-Pozo, J., Benjak, A., Stevenson, K., Simpson, V.R., Busso, P., Mcluckie, J., Loiseau, C., Lawton, C., Schoening, J., Shaw, D.J., Piton, J., Vera-Cabrera, L., VelardeFelix, J.S., Mcdermott, F., Gordon, S.V., Coles, T. & Meredith, A.L. 2016. Red squirrels in the British Isles are infected with leprosy bacilli. Science, 354, 744-747.
Bacles, C.F.E., Burczyk, J., Lowe, A.J. & Ennos, R.A. 2005. Historical and contemporary mating patterns in remnant populations of the forest tree Fraxinus excelsior L. Evolution, 59, 979-990.
Bacles, C.E.F., Lowe, A.J. & Ennos, R.A. 2006. Effective seed dispersal across a fragmented landscape. Science, 311, 628.
Baker, K.H. & Hoelzel, A.R. 2013. Evolution of population genetic structure of the British roe deer by natural and anthropogenic processes (Capreolus capreolus). Ecology and Evolution, 31, 89-102.
Ballingall, K.T., McIntyre, A., Lin, Z., Timmerman, N., Matthysen, E., Lurz, P.W., Melville, L., Wallace, A., Meredith, A.L., Romeo, C. & Wauters, L.A. 2016. Limited diversity associated with duplicated class II MHC-DRB genes in the red squirrel population in the United Kingdom compared with continental Europe. Conservation Genetics, 17(5), 1171-1182.
Barratt, E.M., Gurnell, J., Malarky, G., Deaville, R. & Bruford, M.W. 1999. Genetic structure of fragmented populations of red squirrel (Sciurus vulgaris) in the UK. Molecular Ecology, 8, S55-S63.
Baum, D. 2018. Host salmonid specificity of selected pearl mussel populations. Scottish Natural Heritage Research Report No. 972.
Beddows, A.R. 1961. Holcus lanatus L. Journal of Ecology, 49(2), 421-430.
Beierkuhnlein, C., Thiel, D., Jentsch, A., Willner, E. & Kreyling, J. 2011. Ecotypes of European grass species respond differently to warming and extreme drought. Journal of Ecology, 99(3), 703-713.
Bernatchez, L. 2001. The evolutionary history of brown trout (Salmo trutta L.) inferred from phylogenographic, nested clade, and mismatch analyses of mitochondrial DNA variation. Evolution, 55(2), 351-379.
Bischoff, A., Crémieux, L., Smilauerova, M., Lawson, C.S., Mortimer, S.R., Dolezal, J., Lanta, V., Edwards, A.R., Brook, A.J., Macel, M., Leps, J., Steinger, T. & Müller‐Schärer, H. 2006. Detecting local adaptation in widespread grassland species - the importance of scale and local plant community. Journal of Ecology, 94, 1130-42.
Bjedov, I., Obratov-Petković, D., Mišić, D., Šiler, B. & Aleksić, J.M. 2015. Genetic patterns in range-edge populations of Vaccinium species from the central Balkans: implications on conservation prospects and sustainable usage. Silva Fennica, 49(4), 1283.
Blackman, G.E. & Rutter, A.J. 1954. Endymion nonscriptus (L.) Garcke. Journal of Ecology, 42(2), 629-638.
Blockeel, T., Bosquanet, S.D.S., Hill, M.O. & Preston, C.D. eds. 2014. Atlas of British and Irish Bryophytes. Pisces Publications, Newbury.
Bobbink, R., Hicks, K., Galloway, J., Spranger, T., Alkemade, R., Ashmore, M., Bustamante,
M., Cinderby, S., Davidson, E., Dentener, F. Emmett, B., Erisman, J-W., Fenn, M., Gilliam, F., Nordin, A., Pardo, L. & De Vires, W. 2010. Global assessment of nitrogen deposition effects on terrestrial plant diversity: a synthesis. Ecological Applications, 20(1), 30-59.
Botanical Society of Britain & Ireland, 2019. BSBI Distribution Database. https://database.bsbi.org/
Bourret, V., Kent, M. P., Primmer, C. R., Vasemägi, A., Karlsson, S., Hindar, K., McGinnity, P., Verspoor, E., Bernatchez, L. & Lien, S. 2013. SNP‐array reveals genome‐wide patterns of geographical and potential adaptive divergence across the natural range of Atlantic salmon (Salmo salar). Molecular Ecology, 22, 532-551.
Bragazza, L. 2008. A climatic threshold triggers the die‐off of peat mosses during an extreme heat wave. Global Change Biology, 14, 2688-2695.
Bragg, O.M. & Talis, J.H. 2001. The sensitivity of peat-covered upland landscapes. CATENA, 42, 345-360.
Braithwaite, M.E., Ellis, R.W. & Preston, C.D. 2006. Change in the British Flora 1987-2004. Botanical Society of the British Isles, London.
Burbaité, L. & Csányi, S. 2010. Red deer population and harvest changes in Europe. Acta Zoologica Lituanica, 20, 179-188.
Buyck, B., Hofstetter,V. & Olariaga, I. 2016. Setting the record straight on North American Cantharellus. Cryptogamie. Mycologie, 37, 405-417.
Caporn, S.J.M., Rosenburgh, A.E., Keightley, A.T., Hinde, S.L., Riggs, J.L., Buckler, M. & Wright, N.A. 2018. Sphagnum restoration on degraded blanket and raised bogs in the UK using micropropagated source material: a review of progress. Mires and Peat, 20, 1-17.
Cauwelier, E., Stewart, D.C., Millar, C.P., Gilbey, J. & Middlemas, S.J. 2018. Across rather than between river genetic structure in Atlantic salmon Salmo salar in north‐east Scotland, UK: potential causes and management implications. Journal of Fish Biology, 92, 607-620.
Cauwelier, E., Verspoor, E., Tarr, E.C., Thompson, C. & Young, M. 2009. Genetic diversity and differentiation of freshwater pearl mussel (Margaritifera margaritifera) populations in the UK. Scottish Natural Heritage Commissioned Report No. 344.
Cavers, S. & Cottrell, J.E. 2015. The basis of resilience in forest tree species and its use in adaptive forest management in Britain. Forestry, 88, 13-26.
Charman, T.G., Sears, J., Bourke, A.F.G. & Green, R.E. 2009. Phenology of Bombus distinguendus in the Outer Hebrides. The Glasgow Naturalist, 25 (supplement), 35-42.
Charman, T.G., Sears, J., Green, R.E. & Bourke, A.F.G. 2010. Conservation genetics, foraging distance and nest density of the scarce great yellow bumblebee (Bombus distinguendus). Molecular Ecology, 19, 2661-2674.
Coppins, A. & Coppins, B. 2010. Atlantic hazel. Scottish Natural Heritage, Inverness.
Cosgrove, P., Watt, J., Hastie, L., Sime, I., Shields, D., Cosgrove, C., Brown, L. & Bao, M. 2016. The status of the freshwater pearl mussel Margaritifera margaritifera in Scotland: extent of change since 1990s, threats and management implications. Biodiversity and Conservation, 25(11), 2093-2112.
Cottrell, J.E., Munro, R.C., Tabbener, H.E., Gillies, A.C.M., Forrest, G.I., Deans, J.D. &
Lowe, A.J. 2002. Distribution of chloroplast DNA variation in British oaks (Quercus robur and Q. petraea): the influence of postglacial colonisation and human management. Forest Ecology Management, 156, 181-196.
Coughlan, J., McGinnity, P., O’Farrell, B., Dillane, E., Diserud, O., de Eyto, E., Farrell, K., Whelan, K., Stet, R.J.M. & Cross, T.F. 2006. Temporal variation in an immune response gene (MHC I) in anadromous Salmo trutta in an Irish river before and during aquaculture activities. ICES Journal of Marine Science, 63, 1248-1255.
Dahlberg, A., Genney, D.R. & Heilmann-Clausen, J. 2010. Developing a comprehensive strategy for fungal conservation in Europe: current status and future needs. Fungal Ecology, 3, 50-64.
De Vitis, M., Abbandonato, H., Dixon, K., Laverack, G., Bonomi, C. & Pedrini, S. 2017. The European native seed industry: characterization and perspectives in grassland restoration. Sustainability, 9(10), 1682.
Department for Environment, Food and Rural Affairs, 2019. Conserving our ash trees and mitigating the impacts of pests and diseases of ash: A vision and high-level strategy for ash research.
Donnelly, K., Cavers, S., Cottrell, J.E. & Ennos, R.A. 2018. Cryptic genetic variation and adaptation to waterlogging in Caledonian Scots pine, Pinus sylvestris L. Ecology and Evolution, 8, 8665-8675.
Duguid, R.A., Ferguson, A. & Prodöhl, P. 2006. Reproductive isolation and genetic differentiation of ferox trout from sympatric brown trout in Loch Awe and Loch Laggan, Scotland. Journal of Fish Biology, 69, 89-114.
Eaton, M.A., Brown, A.F., Noble, D.G., Musgrove, A.J., Hearn, R.D., Aebischer, N.J., Gibbons, D.W., Evans, A. & Greory, R.D. 2009. Birds of conservation concern 3: the population status of birds in the United Kingdom, Channel Islands and Isle of Man. British Birds, 102, 296-341.
Eckert, C.G., Samis, K.E. & Lougheed, S.C. 2008. Genetic variation across species’ geographical ranges: the central–marginal hypothesis and beyond. Molecular Ecology, 17(5), 1170-1188.
Edwards T. & Kenyon, W. 2013. Wild deer in Scotland. SPICe Briefing 17/74.
Ennos, R.A., Sinclair, W.T. & Perks, M.T. 1997. Genetic insights into the evolution of Scots pine, Pinus sylvestris L., in Scotland. Botanical Journal of Scotland, 49, 257-265.
Ferguson, A. 2006. Genetics of sea trout, with particular reference to Britain and Ireland. In:
G. Harris & N. Milner. eds. 2006. Sea Trout: Biology, Conservation and Management. Blackwell Publishing, Oxford. pp. 157-182.
Ferguson, A. 2007. Genetic impacts of stocking on indigenous brown trout populations.
Environment Agency Science Report: SC040071/SR, Environment Agency, Bristol. 93pp.
Ferguson, A., Reed, T.E., Cross, T.F., McGinnity, P. & Prodöhl, P.A. 2019. Anadromy, potamodromy and residency in brown trout Salmo trutta: the role of genes and the environment. Journal of Fish Biology, 95, 692-718.
Ferroglio, E., Gortázar, C. & Vicente, J. 2011. Wild ungulate diseases and the risk for livestock and public health. In: R. Putman, M. Apollonio & R. Andersen, eds. Ungulate Management in Europe: Problems and Practices. Cambridge University Press, Cambridge. pp. 192-214.
Forrest, A. 2006. Hybridization in sub-arctic willow scrub in Scotland. PhD, University of Edinburgh.
Gallego-Sala, A.V. & Prentice, I.C. 2013. Blanket peat biome endangered by climate change. Nature Climate Change, 3, 152-155.
Gaudig, G., Fengler, F., Krebs, M., Prager, A., Schulz, J., Wichmann, S. & Joosten, H. 2014. Sphagnum farming in Germany-a review of progress. Mires and Peat, 13(8), 1-11.
Genney, D.R., Coppins, A., Thompson, R., Grundy, K.C. & Gray Stephens, G. 2016. Hazel gloves. In: M. Gaywood, P. Boon, D. Thompson & I. Strachan, eds. 2016. The Species Action Framework Handbook. Scottish Natural Heritage, Battleby, Perth.
Gerber, S., Chadœuf, J., Gugerli, F., Lascoux, M., Buiteveld, J., Cottrell, J., Dounavi, A.,
Fineschi, S., Forrest, L.L., Fogelqvist, J., Goicoechea, P.G., Jensen, J.S., Salvini, D., Vendramin, G.G. & Kermer, A. 2014. High rates of gene flow by pollen and seed in oak populations across Europe. PLoS ONE, 9(1), e85130.
Gilbey, J., Cauwelier, E., Coulson, M.W., Stradmeyer, L., Sampayo, J.N., Armstrong, A.,
Verspoor, E., Corrigan, L., Shelley, J. & Middlemas, S. 2016. Accuracy of assignment of Atlantic salmon (Salmo salar L.) to rivers and regions in Scotland and Northeast England based on single nucleotide polymorphism (SNP) Markers. PLoS ONE, 11(10), e0164327.
Gilbey, J., Cauwelier, E., Sampayo, J., Matejusova, I., Allan, C., Graham, J., Stradmeyer, L. & Middlemas, S. 2018. Identification of the farm of origin of Atlantic salmon smolt escapees in a freshwater Scottish loch using single-nucleotide polymorphic markers. ICES Journal of Marine Science, 75, 2182-2192.
Gimingham, C. 1960. Calluna, a monotypic genus. British Ecological Society, 48, 455-483.
Goulson, D. 2003. Bumblebees: their Behaviour and Ecology. Oxford University Press, Oxford.
Goulson, D., Hanley, M.E., Darvill, B. & Ellis, J.S. 2006. Biotope associations and the decline of bumblebees (Bombus spp.). Journal of Insect Conservation, 10, 95-103.
Graham, J., Marshall, B. & Squire, G.R. 2003. Genetic differentiation over a spatial environmental gradient in wild Rubus ideaus populations. New Phytologist, 157, 667-675.
Graham, J. & McNicol, R.J. 1995. An examination of the ability of RAPD markers to determine the relationships within and between Rubus species. Theoretical and Applied Genetics, 90, 1128-1132.
Grundy, K. 2014. Ecology of Hypocreopsis rhododendri. PhD, University of Aberdeen.
Grundy, K.C., Woodward, S., Genney, D.R. & Taylor, A.F.S. 2012. A molecular approach to explore the extent of the threatened fungus Hypocreopsis rhododendri within wood. Fungal Biology, 116, 354-362.
Haskell, G. 1960. The wild raspberry in Britain. Watsonia, 4, 238-255.
Hatfield, R., Jepsen, S., Thorp, R., Richardson, L. & Colla, S. 2016. Bombus distinguendus. The IUCN Red List of Threatened Species 2016: e.T13340348A46440151.
Hayhow, D.B., Benn, S., Stevenson, A., Stirling-Aird, P.K. & Eaton, M.A. 2017. Status of Golden Eagle Aquila chrysaetos in Britain in 2015. Bird Study, 64(3), 281-294.
Heuertz, M., Fineschi, S., Anzidei, M., Pastorelli, R., Salvini, D., Paule, L., FrascariaLacoste, N., Hardy, O.J., Vekemans, X. & Vendramin, G.G. 2004. Chloroplast DNA variation and postglacial recolonization of common ash (Fraxinus excelsior L.) in Europe. Molecular Ecology, 13(11), 3437-3452.
Hoban, S., Kallow, S. & Trivedi, C., 2018. Implementing a new approach to effective conservation of genetic diversity, with ash (Fraxinus excelsior) in the UK as a case study. Biological Conservation, 225, 10-21.
Höglund, J., Wang, B., Axelsson, T. & Quintela, M. 2013. Phylogeography of willow grouse (Lagopus lagopus) in the Arctic: taxonomic discordance as inferred from molecular data. Biological Journal of the Linnean Society, 110(1), 77-90.
Jackson, F.L., Fryer, R.J., Hannah, D.M., Millar, C.P. & Malcolm, I.A. 2018. A spatiotemporal statistical model of maximum daily river temperatures to inform the management of Scotland's Atlantic salmon rivers under climate change. Science of the Total Environment, 612, 1543-1558.
Jennings, D.L. 1964. Some evidence of population differentiation in Rubus idaeus L. New Phytologist, 63(2), 153-157.
Karlin, E.F., Gardner, G.P., Lukshis, K., Boles, S. & Shaw, A.J. 2010. Allopolyploidy in Sphagnum mendocinum and S. papillosum (Sphagnaceae). The Bryologist, 113(1), 114120.
Karlsson, S., Larsen, B.M. & Hindar, K. 2014. Host‐dependent genetic variation in freshwater pearl mussel (Margaritifera margaritifera L.). Hydrobiologia, 735, 179-190.
Kenward, R.E., Hodder, K.H., Rose, R.J., Walls, C.A., Parish, T., Holm, J.L., Morris, P.A., Walls, S.S. & Doyle, F.I. 1998. Comparative demography of red squirrels (Sciurus vulgaris) and grey squirrels (Sciurus carolinensis) in deciduous and conifer woodland. Journal of Zoology, 244, 7-21.
Kitchener, A.C., Breitenmoser-Würsten, C., Eizirik, E., Gentry, A., Werdelin, L., Wilting, A., Yamaguchi, N. & Johnson, W.E. 2017. A revised taxonomy of the Felidae: The final report of the Cat Classification Task Force of the IUCN Cat Specialist Group. Cat News.
Klemetsen, A., Amundsen, P.A., Dempson, J.B., Jonsson, B., Jonsson, N., O’Connell, M.F. & Mortensen, E. 2003. Atlantic salmon Salmo salar L., brown trout Salmo trutta L. and Arctic charr Salvelinus alpinus L., a review of aspects of their life histories. Ecology of Freshwater Fish, 12, 1-59.
Kohn, D.D., Hulme, P.E., Hollingsworth, P.M. & Butler, A. 2009. Are native bluebells (Hyacinthoides non-scripta) at risk from alien congenerics? Evidence from distributions and co-occurrence in Scotland. Biological Conservation, 142(1), 61-74.
Kohn, D.D., Ruhsam, M., Hulme, P.E., Barrett, S.C.H. & Hollingsworth, P.M. 2019. Paternity analysis reveals constraints on hybridization potential between native and introduced bluebells (Hyacinthoides). Conservation Genetics, 20(3), 571-584.
Kozma, R., Rödin-Mörch, P. & Höglund, J. 2019. Genomic regions of speciation and adaptation among three species of grouse. Scientific Reports, 9, 812.
Langley, P.J.W. & Yalden, D.W. 1977. The decline of the rarer carnivores in Great Britain during the nineteenth century. Mammal Review, 7, 95-116.
Li, P., Holden, J., Irvine, B. & Grayson, R. 2016. PESERA‐PEAT: a fluvial erosion model for blanket peatlands. Earth Surface Processes and Landforms, 41(14), 2058-2077.
Lopes‐Lima, M., Sousa, R., Geist, J., Aldridge, D.C. et al. 2017. Conservation status of freshwater mussels in Europe: State of the art and future challenges. Biological Reviews, 92, 572-607.
Lowday, J.E. & Marrs, R.H. 1992. Control of bracken and the restoration of heathland. III. Bracken litter disturbance and heathland restoration. Journal of Applied Ecology, 29, 212217.
Ludwig, S.C., Aebischer, N.J., Bubb, D., Richardson, M., Roos, S., Wilson, J.D. & Baines, D. 2018. Population responses of Red Grouse Lagopus lagopus scotica to expansion of heather Calluna vulgaris cover on a Scottish grouse moor. Avian Conservation and Ecology, 13(2), 14.
Lusby, J.J. & McNicol, R.J. 1995. Gene flow from cultivated to wild raspberries in Scotland: developing a basis for risk assessment for testing and deployment of transgenic cultivars. Theoretical and Applied Genetics, 90(7-8), 1133-1137.
Macel, M., Lawson, C.S., Mortimer, S.R., Šmilauerova, M., Bischoff, A., Crémieux, L., Doležal, J., Edwards, A.R., Lanta, V., Bezemer, T.M. & van der Putten, W.H. 2007. Climate vs. soil factors in local adaptation of two common plant species. Ecology, 88(2), 424-433.
Mahy, G., Ennos, R.A. & Jacquemart, A-L. 1999a. Allozyme variation and genetic structure of Calluna vulgaris (heather) populations in Scotland: the effect of postglacial recolonization. Heredity, 82(6), 654.
Mahy, G., Vekemans, X. & Jacquemart, A.L. 1999b. Patterns of allozymic variation within Calluna vulgaris populations at seed bank and adult stages. Heredity, 82(4), 432.
Marriott, R., Mchaffie, H. & Mardon, D. 2016. Woolly willow. In: M.J. Gaywood, P. Boon, D. Thompson, & I. Strachan, eds. 2016. Species Action Framework Handbook. Scottish Natural Heritage, Battleby, Perth.
Marrs, R.H., Phillips, J.D.P., Todd, P.A., Ghorbani, J. & Le Duc, M.G. 2004. Control of Molinia caerulea on upland moors. Journal of Applied Ecology, 41(2), 398-411.
Martínez-Padilla, J., Redpath, S.M., Zeineddine, M. & Mougeot, F. 2014. Insights into population ecology from long-term studies of red grouse Lagopus lagopus scoticus. Journal of Animal Ecology, 83, 85-98.
Maskell, L., Henrys, P., Norton, L., Smart, S. & Wood, C. 2013. Distribution of ash Trees
(Fraxinus excelsior) in Countryside Survey Data. Centre for Ecology and Hydrology, Wallingford, UK.
McInnes, C.J., Wood, A.R., Thomas, K., Sainsbur, A.W., Gurnell, J., Dein, F.J. & Nettleton, P.F. 2006. Genomic characterization of a novel poxvirus contributing to the decline of the red squirrel (Sciurus vulgaris) in the UK. Journal of General Virology, 87, 2115-2125.
McKeown, N.J., Hynes, R.A., Duguid, R.A., Ferguson, A. & Prodöhl, P.A. 2010.
Phylogeographic structure of brown trout Salmo trutta in Britain and Ireland: glacial refugia, postglacial colonization and origins of sympatric populations. Journal of Fish Biology, 76, 319-347.
McKinnell, J.M., O’Brien, D., Seymour, T. & Gent, T. 2016. Great crested newt. In: M.J. Gaywood, P. Boon, D. Thompson, & I. Strachan, eds. 2016. Species Action Framework Handbook. Scottish Natural Heritage, Battleby, Perth.
Meharg, A.A., Cumbes, Q.J. & Macnair, M.R. 1993. Pre-adaptation of Yorkshire fog, Holcus lanatus L. (Poaceae) to arsenate tolerance. Evolution, 47(1), 313-316.
Meyer-Lucht, Y., Mulder, K.P., James, M.C., McMahon, B.J., Buckley, K., Piertney, S.B. & Höglund, J. 2016. Adaptive and neutral genetic differentiation among Scottish and endangered Irish red grouse (Lagopus lagopus scotica). Conservation Genetics, 17, 615630.
Minting, P.J. 2016. Common frog. In: C.J. McInerny, P.J. Minting, C. Cathrine & D. O'Brien. 2016. The Amphibians and Reptiles of Scotland. Glasgow Natural History Society.
Mitchell, R.J., Bailey, S., Beaton, J.K., Bellamy, P.E., Brooker, R.W., Broome, A. et al. 2014. The Potential Ecological Impact of Ash Dieback in the UK. JNCC Report No. 483 Joint Nature Conservation Committee, Peterborough, UK.
Mitchell, R.J., Rose, R.J. & Palmer, S.C. 2008. Restoration of Calluna vulgaris on grassdominated moorlands: the importance of disturbance, grazing and seeding. Biological Conservation, 141(8), 2100-2111.
Moore, I., Dodd, J.A., Newton, M., Bean, C.W., Lindsay, I., Jarosz, P. & Adams, C.E. 2018. The influence of aquaculture unit proximity on the pattern of Lepeophtheirus salmonis infection of anadromous Salmo trutta populations on the Isle of Skye, Scotland. Journal of Fish Biology, 92, 1849-1865.
Muir, A.P., Biek, R., Thomas, R. & Mable, B.K. 2014. Local adaptation with high gene flow: temperature parameters drive adaptation to altitude in the common frog (Rana temporaria). Molecular Ecology, 23(3), 561-574.
Muir, A.P., Thomas, R., Biek, R. & Mable, B.K. 2013. Using genetic variation to infer associations with climate in the common frog, Rana temporaria. Molecular Ecology, 22(14), 3737-3751.
Nebel, C., Gamauf, A., Haring, E., Segelbacher, G., Villers, A. & Zachos, F.E. 2015. Mitochondrial DNA analysis reveals Holarctic homogeneity and a distinct Mediterranean lineage in the Golden eagle (Aquila chrysaetos). Biological Journal of the Linnean Society, 116, 328-340.
Norton, L.R., Murphy, J., Reynolds, B., Marks, S. & Mackey, E.C. 2009. Countryside Survey: Scotland Results from 2007. NERC/Centre for Ecology & Hydrology, The Scottish Government, Scottish Natural Heritage, 83pp.
O’Brien, C.D. 2015. Sustainable drainage system (SuDS) ponds in Inverness, UK and the favourable conservation status of amphibians. Urban Ecosystems, 18(1), 321-331.
O’Brien, D., Miró, A., Hall, J., Rae, M., Falaschi, M., Shand, A. & Jehle, R. 2017. Amphibians and people share the benefits of Sustainable Urban Drainage (SuDS) ponds in a fastdeveloping city. Poster at Societas Europaea Herpetologica 19th European Congress of Herpetology; Salzburg, Austria.
Ogden, R., Heap, E., McEwing, R., Tingay, R. & Whitfield, D.P. 2015. Population structure and dispersal patterns in Scottish Golden Eagles Aquila chrysaetos revealed by molecular genetic analysis of territorial birds. Ibis, 157, 837-848.
Palm, S., Laikre, L., Jorde, P.E. & Ryman, N. 2003. Effective population size and temporal genetic change in stream resident brown trout (Salmo trutta, L.). Conservation Genetics, 4(3), 249-264.
Pérez-Espona, S., Goodall-Copestake, W.P., Savirina, A., Bobovika, J., Molina-Rubio, C. & Pérez-Barbería, F.J. 2019. First assessment of MHC diversity assessment in wild Scottish red deer populations. European Journal of Wildlife Research, 65, 22.
Pérez-Espona, S., Pérez-Barbería, F.J., Goodall-Copestake, W.P., Gordon, I.J., Jiggins,
C.D. & Pemberton, J.M. 2009a. Genetic diversity and population structure of Scottish Highland red deer (Cervus elaphus) populations: a mitochondrial survey. Heredity, 102, 199210.
Pérez-Espona, S., Pemberton, J.M. & Putman, R. 2009b. Red and sika deer in the British Isles, current management issues and management policy. Mammalian Biology, 74, 247262.
Pérez-Espona, S., Pérez-Barbería, F.J., McLeod, J., Gordon, I.J., Jiggins, C.D. & Pemberton J.M. 2008. Landscape features affect gene flow of Scottish Highland red deer (Cervus elaphus). Molecular Ecology, 17, 981-996.
Piertney, S.B., MacColl, A.D.C., Bacon, P.J. & Dallas, J.F. 1998. Local genetic structure in red grouse (Lagopus lagopus): evidence from microsatellite DNA markers. Molecular Ecology, 7, 1645-1654.
Piertney, S.B., MacColl, A.D., Bacon, P.J., Racey, P.A., Lambin, X. & Dallas, J.F. 2000. Matrilineal genetic structure and female‐mediated gene flow in red grouse (Lagopus lagopus scoticus): an analysis using mitochondrial DNA. Evolution, 54(1), 279-289.
Pilz, D., Norvell, L., Danell, E. & Molina, R. 2003. Ecology and management of commercially harvested chanterelle mushrooms. U.S. Department of Agriculture, Forest Service, Pacific Northwest Research Station. 83 p.
Piotrowska, M.J., Riddell, C., Hoebe, P.N. & Ennos, R.A. 2018. Planting exotic relatives has increased the threat posed by Dothistroma septosporum to the Caledonian pine populations of Scotland. Evolutionary Applications, 11, 350-363.
Plantlife, 2017. We need to talk about nitrogen. Plantlife, Salisbury.
Price, S.J., Leung, W.T., Owen, C.J., Puschendorf, R., Sergeant, C., Cunningham, A.A., Balloux, F., Garner, T.W. & Nichols, R.A. 2019. Effects of historic and projected climate change on the range and impacts of an emerging wildlife disease. Global Change Biology, 25, 2648-2660.
Prodöhl, P.A, Ferguson, A, Bradley, C.R., Ade, R., Roberts, C., Keay, E.J., Costa, A.R. & Hynes, R. 2019. Impacts of acidification on brown trout Salmo trutta populations and the contribution of stocking to population recovery and genetic diversity. Journal of Fish Biology, 95, 719-742.
Quintela, M., Berlin, S., Wang, B. & Höglund, J. 2010. Genetic diversity and differentiation among Lagopus lagopus populations in Scandinaviva and Scotland: evolutionary significant units confirmed by SNP markers. Molecular Ecology, 19, 2380-2393.
Rasmont, P., Franzén, M., Lecocq, T., Lecocq, T., Harpke, A., Roberts, S.P.M., Biemeijer,
J.C., Castro, L., Cederberg, B., Dvořák, L., Fitzpatrick, Ú., Gonseth, Y., Haubruge, E., Mahé,
G., Manino, A., Michez, D., Neumayer, J., Ødegaard, F., Paukkunen, J., Pawlikowski, T., Potts, S.G., Reemer, M., Settele, J., Straka, J. & Schweiger, O. 2015. Climatic Risk and Distribution Atlas of European Bumblebees. Biorisk 10 (Special Issue), 246 pp.
Rendell, S. & Ennos, R.A. 2002. Chloroplast DNA diversity in Calluna vulgaris (heather) populations in Europe. Molecular Ecology, 11(1), 69-78.
Rich, T.C.G. & Jermy, A.C. eds. 1998. Plant Crib 1998. Botanical Society of the British Isles, London.
Ritchie, J. 1956. Vaccinium myrtillus. Journal of Ecology, 44, 291-299.
Robertson, G.S., Aebischer, N.J. & Baines, D. 2017. Using harvesting data to examine temporal and regional variation in Red Grouse abundance in the British uplands. Wildlife Biology, 2017(4),
Robertson, P.A., Park K.J. & Barton, A.F. 2001. Loss of heather Calluna vulgaris moorland in the Scottish uplands: the role of Red Grouse Lagopus lagopus scoticus management. Wildlife Biology, 7, 11-16.
Sainsbury, K.A., Shore, R.F., Schofield, H., Croose, E., Campbell, R.D. & McDonald, R.A. 2019. Recent history, current status, conservation and management of native mammalian carnivores in Great Britain. Mammal Review, 49, 171-188.
Salmela, M. 2011. Adaptive genetic variation in Scots pine (Pinus sylvestris) in Scotland. PhD, University of Edinburgh.
Sanz, N. 2018. Phylographic history of brown trout. In: J. Lobón-Cerviá & N.N. Sanz, eds.
2018. Brown Trout Biology, Ecology and Management. Wiley, Chichester UK. pp. 17-63.
Scottish Natural Heritage, 2016. Deer Management in Scotland: Report to the Scottish
Government from Scottish Natural Heritage 2016. Scottish Natural Heritage, Inverness.
Scottish Montane Willow Group, 2005. Biodiversity: Taxonomy, genetics and ecology of subarctic willow scrub. Royal Botanic Garden Edinburgh, Edinburgh.
Senn, H.V., Ghazali, M., Kaden, J., Barclay, D., Harrower, B., Campbell, R.D., Macdonald, D.W. & Kitchener, A.C. 2018. Distinguishing the victim from the threat: SNP‐based methods reveal the extent of introgressive hybridization between wildcats and domestic cats in Scotland and inform future in situ and ex situ management options for species restoration. Evolutionary Applications, 12, 399-414.
Senn, H.V. & Pemberton, J.M. 2009. Variable extent of hybridization between invasive sika (Cervus nippon) and native red deer (C. elaphus) in a small geographical area. Molecular Ecology, 18, 862-76.
Sharp, P.J. & Moss, R. 1981. A comparison of the responses of captive willow ptarmigan (Lagopus lagopus lagopus), red grouse (Lagopus lagopus scoticus), and hybrids to increasing daylengths with observations on the modifying effects of nutrition and crowding in red grouse. General and Comparative Endocrinology, 45(2), 181-188.
Skog, A., Zachos, F.E., Rueness, E.K., Feulner, P.G.D., Mysterud, A., Langvatn, R.,
Lorenzini, R., Hmwe, S.S., Lehoczky, I., Hartl, G.B., Stenseth, N.C. & Jakobsen, K.S. 2009. Phylogeography of red deer (Cervus elaphus) in Europe. Journal of Biogeography, 36, 6677.
Smith, S.L., Senn, H.V., Pérez-Espona, S., Wyman, M.T., Heap, E. & Pemberton, J.M. 2018.
Introgression of exotic Cervus (nippon and canadensis) into red deer (Cervus elaphus) in Scotland and northwest England. Ecology and Evolution, 8, 2122-2134.
Stace, C.A. 2019. New Flora of the British Isles. Fourth edition. Middlewood Green: C & M Floristics.
Stace, C.A., Preston, C.D. & Pearman, D. 2015. Hybrid Flora of the British Isles. Botanical Society of Britain & Ireland, Bristol.
Stevens, C.J., Wilson, J. & McAllister, H.A. 2012. Biological Flora of the British Isles: Campanula rotundifolia. Journal of Ecology, 100, 821-839.
Stevenson, A.C. & Birks, H.J.B. 1995. Heaths and moorland: long-term ecological changes, and interactions with climate and people. In: D.B.A. Thompson, A.J. Hester & M.B. Usher, eds. Heaths and moorland: cultural landscapes. HMSO, Edinburgh. pp. 224-239.
Storch, I. 2007. Grouse: Status Survey and Conservation Action Plan 2006 - 2010. Gland, Switzerland: IUCN and Fordingbridge, UK: World Pheasant Association. 114pp.
Taylor, K., Rowland, A.P. & Jones, H.E. 2001. Molinia caerulea (L.) Moench. Journal of Ecology, 89, 126-144.
Thomas, P.A. 2016. Biological flora of the British Isles: Fraxinus excelsior. Journal of Ecology, 104(4), 1158-1209.
Thorstad, E.B., Todd, C.D., Bjørn, P.A., Gargan, P.G., Vollset, K.W., Halttunen, E., Kålås, S., Uglem, I., Berg, M. & Finstad, B. 2014. Effects of salmon lice on sea trout - a literature review. NINA Report 1044, 162pp.
Tonhasca, A. & Macdonald, M.A. 2016. Great yellow bumblebee. In: M.J. Gaywood, P.J.
Boon., D.B.A. Thompson & I.M. Strachan, eds. 2016. The Species Action Framework Handbook. Scottish Natural Heritage, Battleby, Perth.
Urquhart, K., Pert, C.C., Fryer, R.J., Cook, P., Weir, S., Kilburn, R., McCarthy, U., Simons, J., McBeath, S.J., Matejusova, I. & Bricknell, I.R. 2010. A survey of pathogens and metazoan parasites on wild sea trout (Salmo trutta) in Scottish waters. ICES Journal of Marine Science, 67, 444-453.
Vøllestad, L.A. 2018. Understanding brown trout population genetic structure: A NorthernEuropean perspective. In: J. Lobón-Cerviá, & N.N. Sanz, eds. 2018. Brown Trout Biology, Ecology and Management. Wiley, Chichester UK. pp. 127-144.
Walker, K.J., Hodder, K.H., Bullock, J.M. & Pywell, R.F. 2004a. A review of the potential effects of seed sowing for habitat re-creation on the conservation of intraspecific biodiversity. Defra Contract BD1447.
Walker, K.J., Stevens, P.A., Stevens, D.P., Mountford, J.O., Manchester, S.J. & Pywell, R.F. 2004b. The restoration and re‐creation of species‐rich lowland grassland on land formerly managed for intensive agriculture in the UK. Biological Conservation, 119, 1-18.
Ward, A.I. 2005. Expanding ranges of wild and feral deer in Great Britain. Mammal Review, 35, 165-173.
Watson, J. 2010. The Golden Eagle. Second Edition. London, Poyser.
Watt, J, Cosgrove, P.J & Hastie, L.C. 2015. A national freshwater pearl mussel (Margaritifera margaritifera, L.) survey of Scotland. Scottish Natural Heritage Commissioned Report No. 901.
Welch, D., Scott, D., Moss, R. & Bayfield, N. 1994. Ecology of blaeberry and its management in British moorlands. Grange-over-Sands, Institute of Terrestrial Ecology.
Wenzel, M.A. & Piertney, S.B. 2014. Fine-scale population epigenetic structure in relation to gastrointestinal parasite load in red grouse (Lagopus lagopus scotica). Molecular Ecology, 23, 4256-4273.
Wenzel, M.A., James, M.C., Douglas, A. & Piertney, S.B. 2015a. Genome-wide association and genome partitioning reveal novel genomic regions underlying variation in gastrointestinal nematode burden in a wild bird. Molecular Ecology, 24, 4175-92.
Wenzel, M.A. & Piertney, S.B. 2015b. Digging for gold nuggets: uncovering novel candidate genes for variation in gastrointestinal nematode burden in a wild bird species. Journal of Evolutionary Biology, 28, 807-825.
Wenzel, M.A., Webster, L.M.I., Paterson, S. & Piertney, S.B. 2015c. Identification and characterisation of polymorphic candidate genes for response to parasitic nematode (Trichostrongylus tenuis) infection in red grouse (Lagopus lagopus scotica). Conservation Genetics Resources, 7, 23-28.
Whitehead, G.K. 1964. The deer of Great Britain and Ireland. Routledge & Kegan Paul, London.
Whittet, R., Cavers, S., Ennos, R. & Cottrell J. 2019.Genetic considerations for provenance choice of native trees under climate change in England. Forestry Commission Research Report Forestry Commission, Edinburgh. i–viii + 1–44 pp.
Wilkinson, M.K., Eaton, E.L. & Morison, J.I.L. 2017. Variation in date of bud burst in Quercus robur and Q. petraea across a range of provenances grown in Southern England. European Journal Forest Research, 136, 1-12.
Williams, P.H., Araújo, M.B. & Rasmont, P. 2007. Can vulnerability among British bumblebee (Bombus) species be explained by niche position and breadth? Biological Conservation, 138, 493-505.
Wilson, J.Y., 1959. Vegetative reproduction in the bluebell, Endymion nonscriptus (L.) Garcke. The New Phytologist, 58(2), 155-163.
Worrell, R., Rosque, C. & Ennos, R. 2014. Long-distance colonisation of oak dispersed by jays in Highland Scotland. Scottish Forestry, 68, 24-30
This report should be cited as: Hollingsworth, P.M., O’Brien, D., Ennos, R.A., Ahrends,
A., Ballingall, K.T., Brooker, R.W., Burke, T., Cavers, S., Dawson, I.K., Elston, D.A., Kerr, J.,
Marshall, D.F., Neaves, L., Pakeman, R.J., Trivedi, C., Wall, E., Wright, F., Yahr, R., Bean, C.,
Blake, D., Campbell, R., Comont, R., Finger, A., Fraser, K., Genney, D., Hall, J., Hannah, A.,
Jehle, R., Jones, S., Kohn, D., Llewellyn, M., Lurz, P., Macdonald, I., McIntosh, J., Mitchell, R.,
O’Dell, J., Page, S., Pemberton, J., Pérez-Espona, S., Piertney, S., Sime, I., Thompson, D.,
Ogden, R. 2020. Scotland’s Biodiversity Progress to 2020 Aichi Targets: Aichi Target 13 – Genetic Diversity Maintained – Supplementary Report 2020. Inverness, Scottish Natural Heritage.
ISBN 978-1-78391-952-9
Disclaimer: Scottish Natural Heritage (SNH) has changed its name to NatureScot as of the 24th August 2020.
At the time of publishing, this document may still refer to Scottish Natural Heritage (SNH) and include the original branding. It may also contain broken links to the old domain.
If you have any issues accessing this document please contact us via our feedback form.