NatureScot Research Report 1259 - A risk-based approach to peatland restoration and peat instability
Year of publication: 2023
Authors: Mills, A.J. and Rushton, D.
Cite as: Mills, A.J. and Rushton, D. 2023. A risk-based approach to peatland restoration and peat instability. NatureScot Research Report 1259.
Keywords
peat; stability; landslides; restoration; risk; protocol; bog; Scotland
Background
This report describes a risk-based approach to assessing the potential impacts of peatland restoration groundworks on peat stability. The approach is delivered as an interactive tool for Peatland ACTION officers to determine potential risks associated with proposed peat restoration activities. The tool is intended to support Peatland ACTION officers in avoiding groundworks on sites with a past history of instability, with high natural susceptibility to instability or which may become more prone to instability in association with some restoration activities. Because restoration is intended to increase the effectiveness of peatlands as carbon stores, it is important that peat landslides and associated carbon losses do not occur as a result of restoration.
The report provides an overview of the physical properties of peat and the characteristics of naturally occurring and human-induced peat landslides. The mechanical effects of peat restoration techniques are considered conceptually and informed by a series of case study site visits to peat restoration sites (including some that have experienced instability following restoration). The risk-based approach is presented as a three stage process: i) determination of the natural or baseline landslide likelihood, ii) determination of the modified landslide likelihood (as a function of restoration groundworks) and iii) as risk calculated in response to the consequences felt by a range of receptors.
Main findings
- Peat instability is a relatively rare phenomenon in Scotland (c. 50 reported failures) and there have been very few instances reported in association with restoration activities. Around 100 restoration areas with post-restoration satellite imagery available were reviewed, and none were found to have evidence of post-restoration instability.
- However, to ensure that appropriate techniques are applied in the context of local site and peat conditions the potential effects of restoration groundworks on peat stability must still be evaluated using both qualitative and quantitative approaches. An approach is provided to enable Peatland ACTION officers to assess baseline stability at a site-scale, identify ‘modified’ stability given selected restoration groundworks and determine risk-based on receptors within, downslope and downstream of proposed restoration sites.
- It should be noted that the focus of this report is on large-scale peat instability, manifest as landslides, and not on other potential geomorphological responses to restoration groundworks, such as erosion or weathering. Issues relating to erosion monitoring and control are already addressed within the good practice guidance for restoration associated with each technique.
Acknowledgements
This report was enhanced by contributions in the field and in workshops by a number of NatureScot staff and affiliated Peatland ACTION officers, in particular Dr Christina Wood, Ewan Campbell, Gear óid Murphy, Emma Stewart, Tim Cockerill, Sue White and Dr Emily Taylor.
1. Introduction
1.1. Background
Peat landslides are well-documented but relatively rare phenomena occurring in upland and lowland peatlands in the UK, Ireland and globally. In the UK and Ireland, pressure to develop peat uplands has generated a range of guidance aimed at assisting developers and stakeholders in ensuring safe, responsible and sustainable development of the valuable carbon stores and unique habitats associated with peat environments (Scottish Government, 2011, 2017; Scottish Government, SNH and SEPA, 2017; Scottish Renewables and SEPA, 2012).
At present, peat landslides (often referred to as peat instability), are one of a range of issues considered in environmental impact assessment for upland energy generation projections, notably wind farms, and the Scottish Government has prepared specific guidance to inform these developments (Scottish Government, 2017). Concurrently, considerable efforts are being made to reclaim peatlands degraded by poor land management practices, including artificial drainage, afforestation and industrial and smaller-scale peat cutting for fuel. In common with groundworks for energy projects, restoration activities may have the potential to reduce the stability of peat, with a single peat landslide event capable of displacing significant volumes of peat and ultimately leading to loss of carbon as the landslide deposits break down. If consequences of instability are high, e.g. pollution of a sensitive watercourse, then the benefits of restoration may be marginal relative to the potential losses associated with a landslide.
As a lead proponent of peatland restoration, NatureScot (NS), through Peatland ACTION, has set over 19,000 ha of degraded peatland on the road to restoration through a variety of techniques, many of which involve rewetting of peatlands in order to restore their hydrological and ecological function. However, isolated occurrences of peat instability at restored sites indicate that restoration activities may have a locally negative effect on peat stability.
For this reason, NS commissioned East Point Geo Ltd (EPG) to identify the potential impacts of restoration activities on peat stability and develop a tool to enable Project Officers to make decisions on the suitability of restoration activities. The tool was to be informed by site conditions, existing technical knowledge of peat instability, and was to consider the full range of benefits and potential risks associated with restoration activities. The resulting risk-based protocol is to be applied by Peatland ACTION Officers when identifying sites and approaches suitable for restoration. The use of ‘risk-based’ in this report refers to the consideration of both landslide consequences and landslide likelihood, rather than limiting focus to the latter.
1.2. Scope of work
In order to develop the risk-based protocol, the following tasks were identified as the scope of work (NS, 2019):
- Review of current perception of risk of peat landslide in areas subject to restoration of degraded peatland.
- Identification of how activities associated with Peatland ACTION restoration can act as triggers for peat mass movement.
- Evaluation of the relevance and effectiveness of mitigation options.
- Development of the peat landslide risk assessment protocol.
- Development of a peat landslide risk assessment recording template.
- Identification of knowledge gaps and peat stability parameters for Peatland ACTION long term monitoring strategy.
Section 1.3 details where these tasks are addressed in the main body of this report.
1.3. Report structure
The risk-based protocol has been informed by a combination of desk study, practitioner liaison, field study and geomorphological and geotechnical analysis. This is documented as follows:
Chapter 2 provides an introduction to peat instability as a phenomenon, informed by primary research undertaken for this study and more widely, and follows with an overview of peatland restoration practice as context to subsequent consideration of impacts.
Chapter 3 evaluates evidence for restoration activities being associated with instability, through a review of Peatland ACTION projects, field-based assessment of specific case study sites with Peatland ACTION officers and wider review of publicly available information.
Chapter 4 introduces qualitative and quantitative approaches to the analysis of baseline (pre-restoration) peat stability and modified (post-restoration) effects and then provides technique-by-technique evaluation of their effects.
Chapter 5 describes the approach to and content of the risk-based protocol for which this report provides the technical background and supporting information. Two protocols have been developed for differing peatland and restoration settings (blanket bogs and raised bogs).
Chapter 6 considers knowledge gaps and identifies monitoring criteria for evaluating the medium and long-term effects of restoration on peat stability. Guidance is provided on revising the risk-based protocols as new restoration techniques become available and as restored sites continue to adjust to their restored states.
The risk-based protocols are supplied as electronic appendices to this report, with additional appendices providing supporting information for their use.
1.4. Project phases and use of the protocols
Due to the nature of the Peatland ACTION funding cycle, the protocols should be applied to a prospective restoration site as early as is practicable. This is because any changes to restoration extent or methodology arising from use of the protocols will directly affect the form and costing of a proposed project, as well as the risks. When approving projects for funding, it is helpful to have the risk levels identified and the restoration techniques verified as appropriate to the ground conditions present. Late application of the protocols, i.e. once a proposal is mature, may lead to a request to revise the restoration approach, slowing down the application process.
2. A review of peat instability and peatland restoration
2.1. Peat soils
This section provides a brief summary of physical and hydrological characteristics of peat as a precursor to a discussion of peat instability as a geomorphological process within peatlands. The summary is intended to provide context to the consideration of the impacts that restoration activities may have on the physical and hydrological structure of peat, and in turn, its stability.
2.1.1. Physical characteristics of peat
Peat is an organic soil comprising the decayed organic matter of wetland plants. Peat accumulates where the rate of deposition of dry vegetative matter exceeds its rate of decay, with physical, chemical and biological processes associated with wetland conditions allowing the decaying organic matter to retain some of its plant structures for very long periods i.e. thousands of years (Warburton, 2014). As an engineering material, it is of low density, high compressibility and initial high permeability, high natural water content, very high organic content and low strength. As a non-mineral soil, the fibrous plant structures and amorphous granular matrix that give peat its character do not lend themselves to standard geotechnical analysis since soil mechanics theory is based on inter-particle frictional behaviour. While there are now specialised geotechnical techniques designed to overcome some of these limitations (Boylan and Long, 2014; Dykes, 2008), these techniques are not commonly used in standard field or laboratory investigations. The strength characteristics of peat are of particular relevance to assessment of peat instability and is considered later in Section 4.
Peat deposits are generally described in terms of their depth and degree of decomposition. The former is usually determined by peat depth probing or coring. During probing, rods are inserted into the ground until the underlying substrate, usually rock or a stiff or granular glacial till, is contacted. While this provides a measure of peat depth, it provides little information on the material characteristics of the peat, which is usually assessed by coring. Cores retrieved from below the ground surface enable peat soils to be visually described and logged using the von Post scale (Edil, 2001, see section 4.3). Typically, peat becomes more decomposed (or humified) with increasing depth, comprising a top vegetative ‘turf’ of living vegetation, an underlayer of partially decomposed (but still recognisable fibrous) plant material, and a basal layer of significantly decomposed peat soil. The physical characteristics of peat of relevance to peat instability are shown conceptually on Figure 2.1.
In summary, peat can be considered as comprising a living turf layer and active root zone – the fibrous layer, an underlying partially to well humified (decomposed) layer – humified peat, and sometimes, at the base of the peat profile a fully humified or decomposed layer – amorphous peat. The relative thicknesses of these layers have a strong part to play in controlling the susceptibility of a peatland to failure and the resulting type of landslide (discussed further below).
2.1.2. Hydrological characteristics of peat
Because the hydrological characteristics of peat are critical to its surface vegetation cover, and in turn, habitat, the layering of peat into an upper acrotelm and lower catotelm is also used to characterise peat deposits. The acrotelm lies above the level of seasonal water table fluctuations and displays active root growth, while the catotelm lies below it, remaining permanently saturated. Visually, the acrotelm typically has the appearance of a thick, fibrous mat with some tensile strength (or resistance to tearing), while the catotelm appears as a soil, with fibres in varying states of decomposition visible throughout the soil matrix.
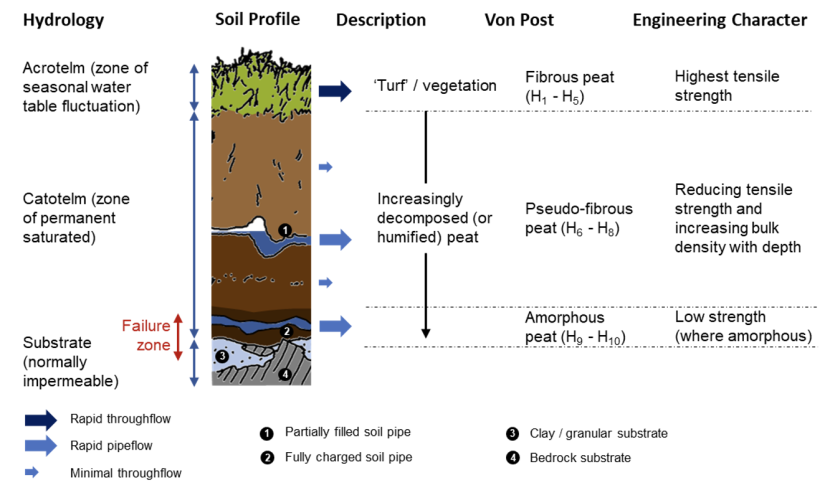
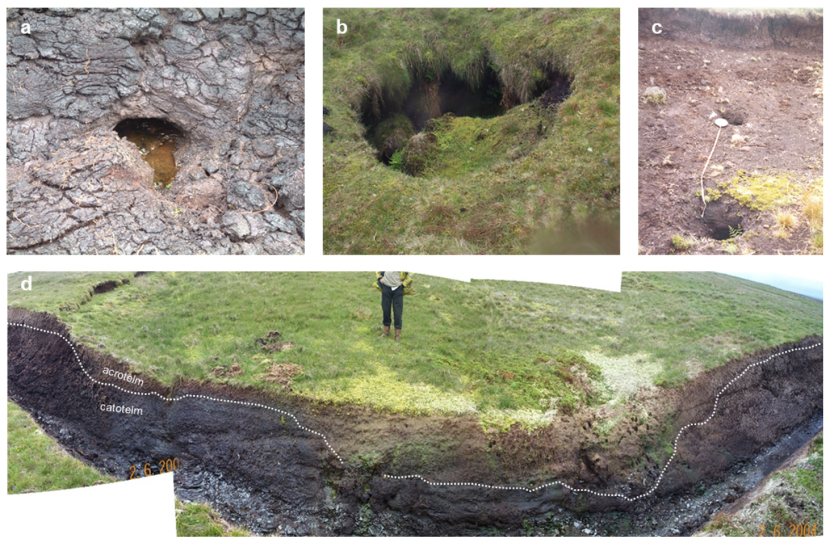
a) pipe outline in bare and dried peat (the pipe is c. 20cm in width), b) collapsed pipe ceiling (c. 1.5m wide), c) collapsed pipe ceilings (c. 20cm wide) on a peat shear surface with mineral debris brought to the surface by artesian water pressure, d) diffuse surface drainage (Sphagnum carpet) with the likely varying depth of catotelm as a function of vegetation (indicated as a hashed line).
The hydrology of peatlands is complex, varying laterally and with depth over short distances. The well-decayed catotelm is relatively impermeable, with limited flow of water through the soil matrix. Instead, the majority of water transfer within the catotelm occurs within natural soil ‘pipes’ which undulate within the peat mass and are typically aligned at boundaries within the peat (e.g. between well decomposed and less well decomposed layers or at the boundary between the peat and underlying substrate). Peat pipes can be observed in peatlands at exposures or where their ceilings have collapsed (see Figure 2.2). As can be seen from the photographs, peat pipes are not normally full of water, however, fully charged pipes are often implicated as potential triggers or preconditions for peat landslides (see Section 2.2.2). Where significant volumes of water are trapped beneath the upper peat soils, the acrotelm and underlying soil may ‘quake’ underfoot, with these localised areas referred to as ‘quaking bog’.
The majority of water movement occurs within the acrotelm. When water tables are low, i.e. at the base of the acrotelm, e.g. during dry summer months, the peat mass has a greater capacity to absorb water before surface overland flow (or ‘runoff’) occurs. Water still moves downslope within the acrotelm, but more slowly. Once the water table is at or near ground surface (typically in winter, though the acrotelm can rewet quickly, even after drought), the peat mass is fully saturated and has no more capacity to absorb water, and runoff can occur quickly after rainfall events, leading to the description of peat covered catchments as ‘flashy’ (Shuttleworth et al., 2019; Holden and Burt, 2003).
2.2. Peat instability
This section provides an overview of peat instability features including their forms (morphology), causes, consequences and significance. As part of this study, a landslide database compiled by Mills (2002) has been updated with reference to satellite imagery in Google Earth that was unavailable at the time of the original research. This imagery has provided an opportunity to validate the original findings and attain a better understanding of site-specific controls of peat instability including slope angle, geomorphological setting and human effects (such as drainage, forestry, peat cutting and construction).
2.2.1. Types of peat instability
Peat instability takes a number of forms, the majority of which can be identified either through site walkover or remotely from high resolution aerial photography or satellite imagery (at or better than 0.15m ground resolution):
- Minor instability: localised and small-scale features that are not generally precursors to major slope failure and that include gully sidewall collapses, pipe ceiling collapses, minor slumping along diffuse drainage pathways (e.g. along flushes); indicators of incipient instability including development of tension cracks, tears in the acrotelm (upper vegetation mat), compression ridges, or bulges / thrusts; these latter features may be warning signs of larger scale major instability (such as landsliding) or may simply represent a longer term response of the hillslope to drainage and gravity, i.e. creep.
- Major instability: comprising various forms of peat landslide, ranging from small scale collapse and outflow of peat filled drainage lines/gullies (occupying a few-10s cubic metres), to medium scale peaty-debris slides in organic soils (10s to 100s cubic metres) to large-scale peat slides and bog bursts (1,000s to 100,000s cubic metres).
Examples of minor and major instability are shown in Figure 2.3 and Figure 2.4 respectively. Typically, major instability is reported in the public domain and is the focus of research, understanding and mitigation efforts when groundworks are undertaken in peatlands.
The occurrence of minor instability within peatlands can be considered alongside other ongoing processes of erosion such as gully erosion or weathering of bare peat faces and surfaces and represents progressive (rather than catastrophic) natural degradation. However, the triggering of major instability, either naturally, or by human activities can have significant effects for peat habitats and adjacent and connected habitats such as watercourses. If restoration groundworks act as triggers for such events, it may be that the negative consequences outweigh the benefits of restoration work.
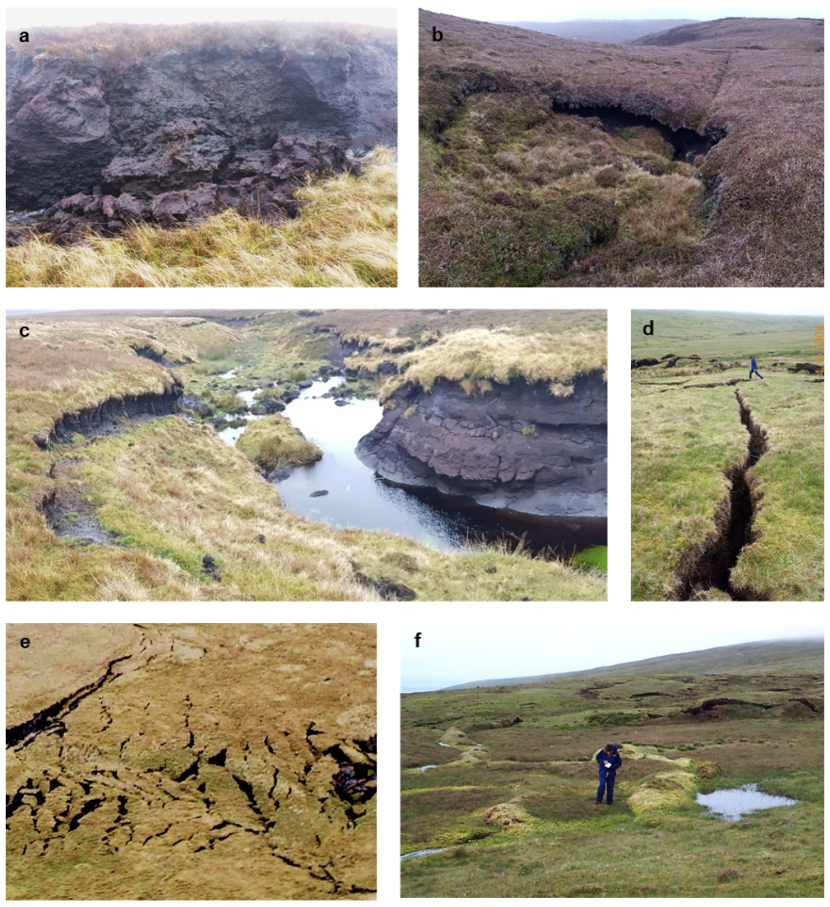
Examples of minor instability: a) gully sidewall collapse (c. 1.25m high), b) pipe ceiling collapse (c. 3m in diameter), c) slumping along a watercourse (bank top to bank top c. 10m), d) tension cracks (c. 30cm wide), e) tears of variable width, typically 0.5-1.0m), f) compression ridges / bulging (c. 1.5m wide in cross-section).
Landslides in peatlands have been reported since the 1600s and have been subject to numerous case study based accounts of their morphology and potential causes. A range of terminology has been used to describe them based on the peatland setting, peat thickness, morphology (or form) and perceived failure mechanism. A useful review was provided by Dykes and Warburton (2007a). They described six types of landslide according to the position of the failure surface within the peat and substrate profile and the composition of the peat (either a ‘two layer’ system of fibrous over amorphous peat, or a ’single layer’ system). These forms can be simplified into three types of failure that summarise their settings, failure mechanism and materials as shown in Figure 2.5 (as per Evans and Warburton, 2007).
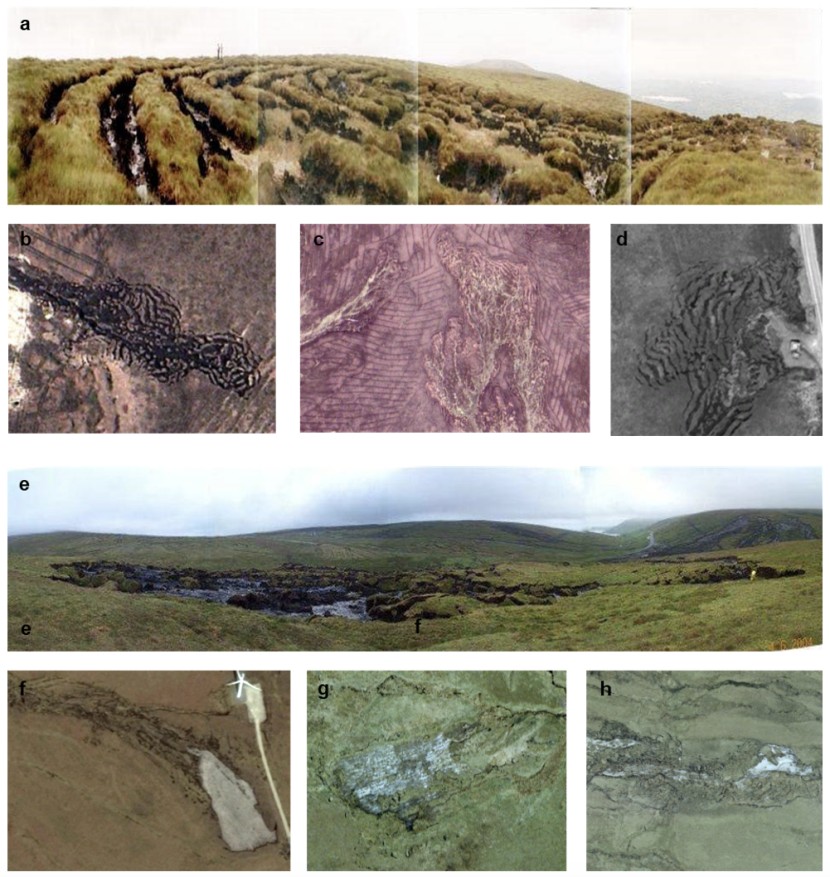
a) to d) are bog bursts and show clear ‘ribbons’ of vegetated peat separated by tears and cracks filled with slurried basal peat; e) to h) are peat slides and show fully exposed mineral substrates (in grey) in the scar area with stranded rafts and blocks of material downslope of the scar.
Referring to Figure 2.5, the key characteristics of the three failure types are as follows:
- Bog burst: very large-scale (usually greater than 10,000m3; Mills, 2002) spreading failures in which the landslide retrogresses (cuts) upslope in ‘slices’ from the point of failure and outflow of slurried peat carries intact blocks downslope. Failure is triggered at the bottom of the slope. Peat is typically deeper (greater than 1.0m and up to 10m) and more amorphous than sites experiencing peat slides, with shallower slope angles (typically 2°-5°). Much of the peat displaced during the event may remain within the initial failure zone. Bog bursts have rarely been reported in Scotland other than in the Western Isles (e.g. Bowes, 1960).
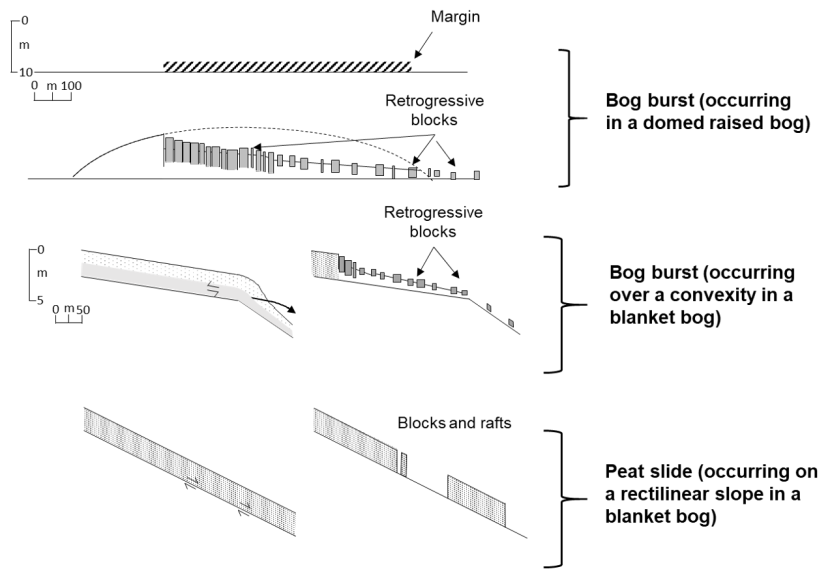
Diagram showing the classification of peat landslides into bog bursts occurring in a domed lowland raised bog, bog bursts occurring over a convexity in a blanket bog, and peat slides occurring on rectilinear slopes on blanket bog.
- Peat slide: medium to large-scale (typically less than 10,000m3; Mills, 2002) landslides in which failure initiates as large rafts of material which subsequently break down into smaller blocks and slurry. Peat slides occur ‘top-down’ from the point of initiation on a slope, in thinner peats than bog bursts (between 0.5m and 1.5m), and on moderate slope angles (typically 5°-15°). Failure is typically triggered at the top of the slope, with the failure plane propagating downslope.
- Peaty-debris slides: small to medium scale (typically less than 10,000m3) landslides similar to peat slides, but in non-peat soils (i.e. <0.5m thick). Failure occurs at the transition zone between peat and organic soil. Peaty-debris slides lack rafting and typically occur ‘top down’ on steeper slopes than peat slides (>10°). They are more common than peat slides and bog bursts and therefore less frequently reported as exceptional events.
This study focuses on minimising the potential for peat slides and bog bursts, since these are the largest features occurring in true peatlands (rather than organic soils). Typical morphological features of these peat slides and bog bursts are shown on Figure 2.6.
Key characteristics of peat slides and bog bursts are shown on Figure 2.7, including the slope angles on which they are sourced, lengths, widths, areas and volumes.
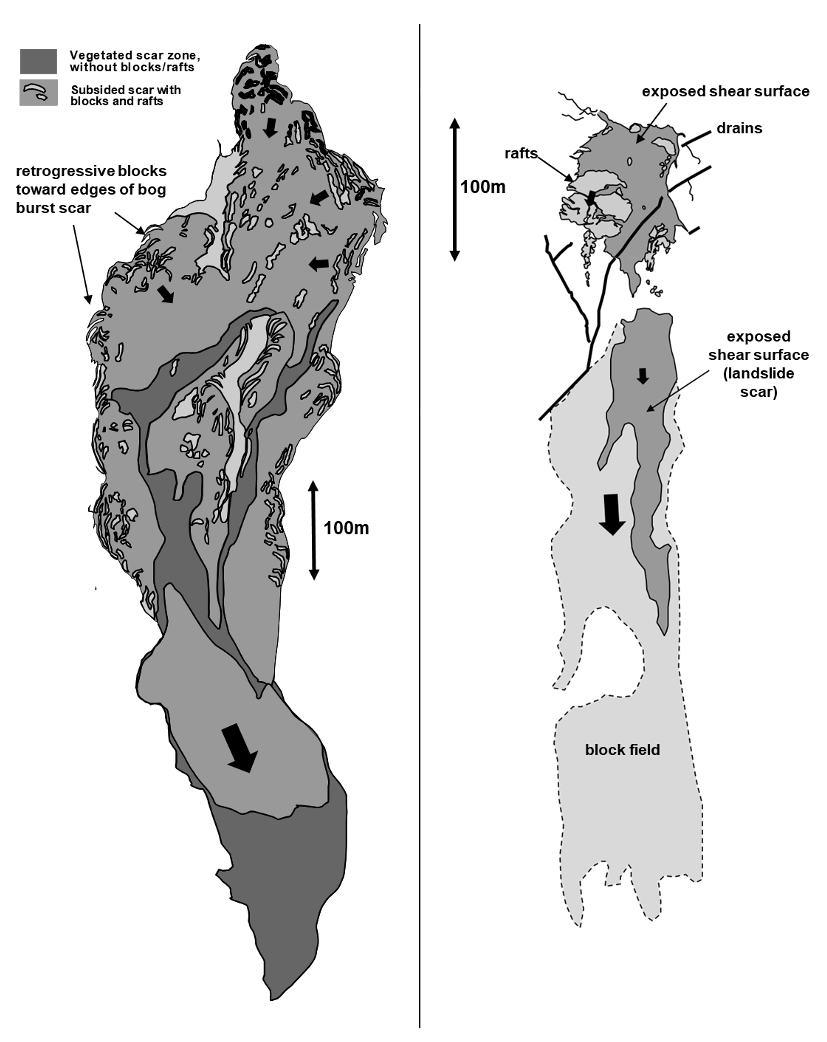
2.2.2. Distribution
While peat landslides have been reported outside the British Isles (notably in Switzerland, Germany, Argentina, Australia and the sub-Antarctic Islands), they have been most frequently documented in the British Isles, with most of the early reports within Ireland and Northern Ireland. As part of this study, a landslide database compiled by Mills (2002) has been updated with new examples published in news articles, academic and grey literature or identified as part of review of satellite imagery for this study. The locations are shown on Figure 2.8.
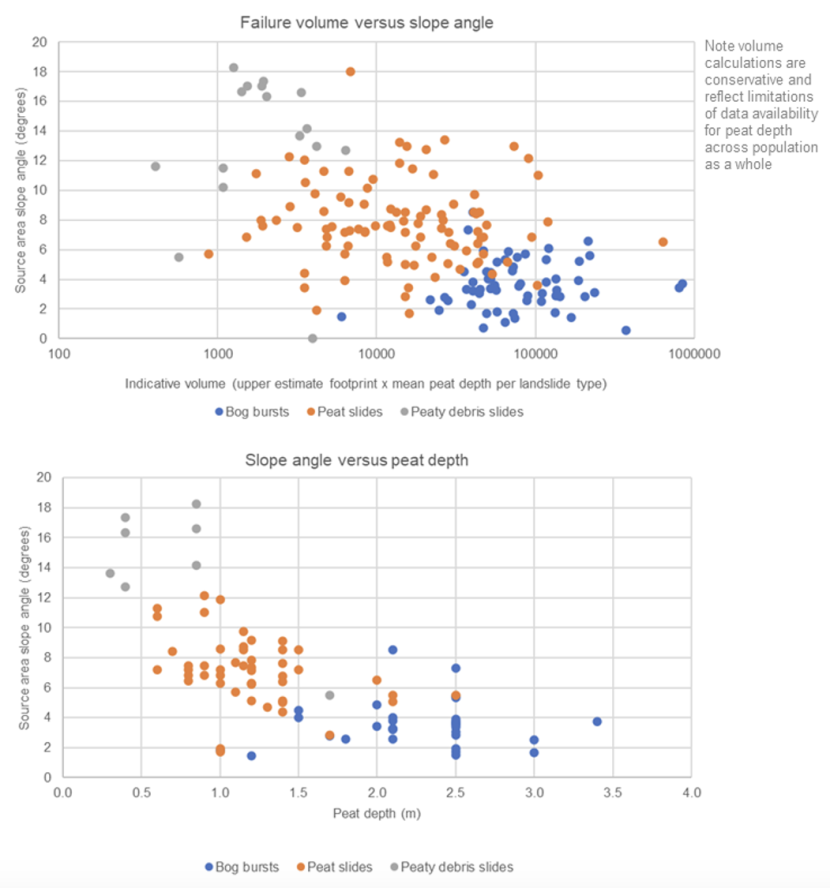
(a) The relationship between slope angle, failure volume and mass movement type (b) The relationship between slope angle, peat depth and mass movement type, (c) Number of peat landslides associated with different slope profiles, (d) Number of landslides associated with orientation of artificial drains, and (e) Number of peat landslides associated with different land uses.
The 2002 database was based primarily on review of secondary sources, informed by field visits to many of the reported failure locations. The update for this report involved revisiting published failure locations and collecting primary data on dimensions, setting, drainage, slope form and land-use. An additional visual review was undertaken within GoogleEarthTM of much of the peatland area within Scotland to determine whether peat landslides were significantly greater in number than reported, however this review, while not exhaustive indicated very little evidence of unreported failures in the main peatland areas reviewed. No such review as undertaken outside Scotland due to the focus of this report.
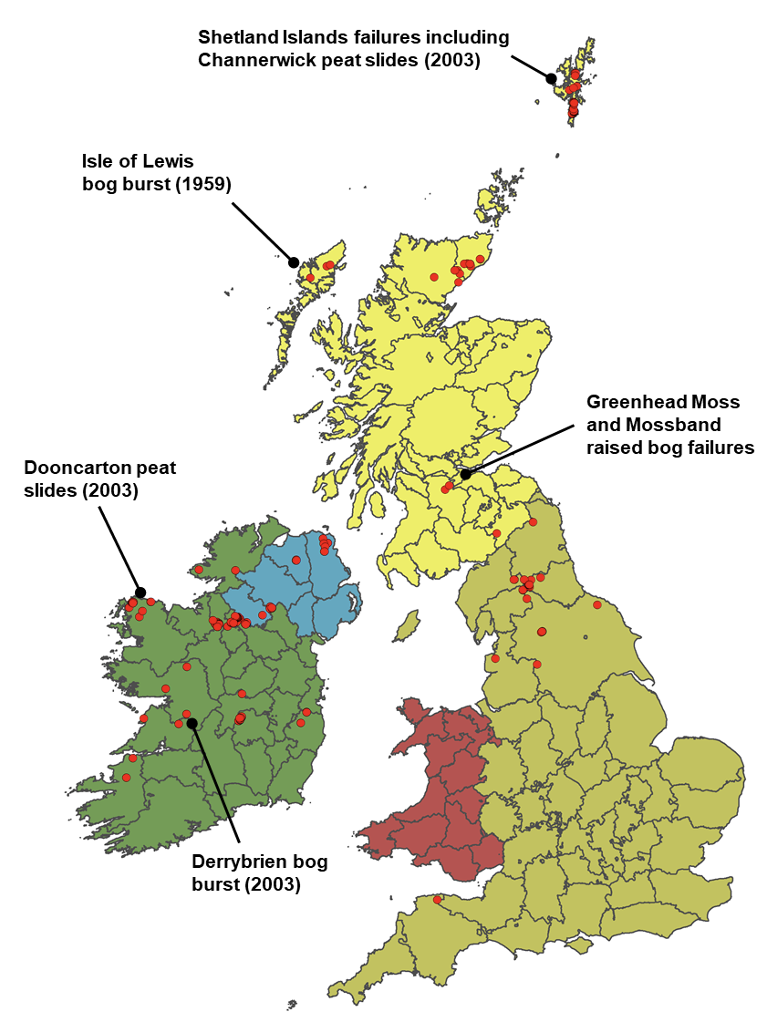
While landslides on the Shetland Islands and Outer Hebrides have been reported in news articles (The Shetland Times, 2015) and published in academic journals (Bowes, 1960; Dykes and Warburton, 2007b), the majority of the landslides shown in mainland Scotland have not been published and have been identified from satellite imagery review (increasing the number of events now in the public domain). These failures are generally concentrated in the northeast Highlands. There is a notable absence of reported failures in the peatlands of Wales. Given the interest in peat mass movements in recent decades, if these failures had occurred, it might be expected that they would have been reported. A systematic review of Welsh peatlands may address this.
A key observation is that landslides in peat are relatively rare occurrences in Scotland, given the extensive peat cover. A second key observation, derived from the updated peat landslide database, is that peat landslides are often spatially clustered, i.e. they occur on the same hillslopes as previous failures (Figure 2.9), though not at the same locations (i.e. they rarely ‘reactivate’).
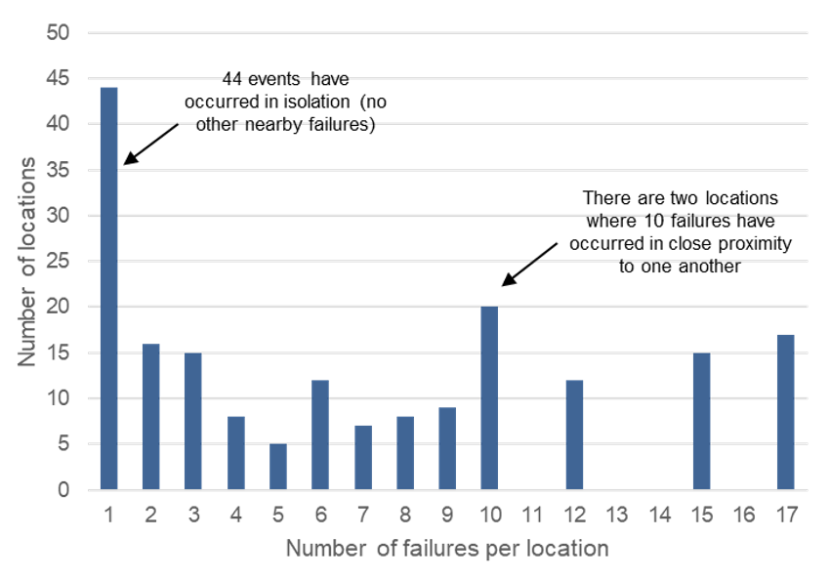
The graph shows that whilst many peat landslide events occur in isolation, there are notable clusters of events that occur in close proximity to previous ones.
This implies that if a peatland already has already experienced peat instability, there may be a higher likelihood of a new event than in nearby areas where no prior peat landslides have occurred. This is logical, since there are likely to be intrinsic physical and hydrological properties that make some peatlands more susceptible to landslides than others.
2.2.3. Historical frequency
Warburton (2015) provides a helpful discussion of the historical frequency of peat landslides as derived from recorded events in the British Isles, noting that increasing reporting of landslides over time may simply reflect the increasing volume and quality of documentary sources and the increasing occupation of rural areas where peat landslides occur. Figure 2.10 shows a frequency curve (aggregated by decade) for reported peat landslides, including those identified in the updated landslide database.
A key observation is that the number of recorded events with known failure dates is relatively low. The full peat landslide database for the British Isles (shown visually on Figure 2.8) indicates in the order of c. 190 individual landslide scars. The proportion of these that are peat slides and bog bursts are shown by country in Table 2.1.
Table 2.1. Proportion of peat landslides by type and country of occurrence
Location |
Peat slides |
Bog bursts |
---|---|---|
Scotland |
51 |
5 |
England |
22 |
2 |
Wales |
0 |
0 |
Ireland and Northern Ireland |
38 |
53 |
Other than in Ireland and Northern Ireland, peat slides are dominant failure mechanism, with roughly 1 in every 10 failures being a bog burst.
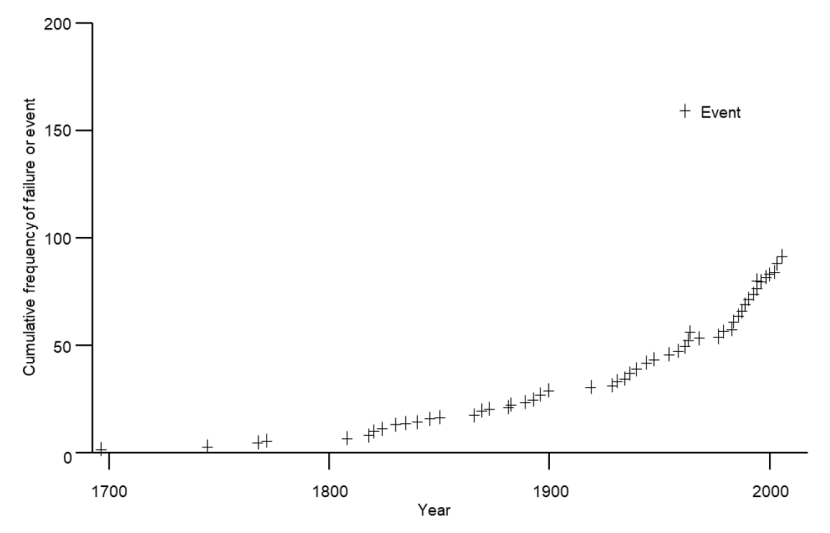
Graph showing the historical frequency of reported peat landslide events. The number of recorded events with known failure dates is relatively low, but overall there is far more recording of events in the last 30 years or so, than previously.
2.2.4. Causes
Landslides are generally considered to occur in response to long term preconditioning factors and triggers. Preconditioning (or preparatory) factors reduce the stability of a slope, while triggers cause the landslide. In relation to restoration, it is important to understand how groundworks may act to precondition slopes or trigger failure.
Table 2.2 details preparatory factors for peat instability hypothesised in research into peat landslides since they were first reported (e.g. Scottish Government, 2017). Many of these preconditions may act in concert.
The effects of restoration activities on these preparatory factors must be considered when evaluating their potential implications for peat landslide risk, particularly given that the effects of restoration may take years or decades to come into effect in modifying peat hydrology and physical properties. This is considered in detail in Section 3.
Table 2.3 details commonly cited triggers for peat instability and provides an indication of where restoration groundworks have the potential to provide a triggering mechanism.
Table 2.2. Preconditions for peat instability
Preparatory factors |
Influence on stability |
---|---|
Increase in mass (peat formation) |
Increase shear stresses acting downslope, long term and progressive where occurring naturally; may occur in the short term where peat spoil is stored on the peat surface |
Increase in mass (water content) |
Increases shear stresses acting downslope, may occur in response to re-wetting e.g. through peat restoration or naturally occurring drain blocking (sidewall collapse) |
Increase in mass (loading by trees) |
Increases shear stresses acting downslope, medium term and may occur where root plates are within peat rather than onto substrate |
Reduction in shear strength |
Reduction in shear strength of peat or substrate through changes in physical structure (either remoulding by progressive creep or vertical fracturing), reduction of frictional contact (e.g. through increasing buoyancy as water table rises), chemical or physical weathering (e.g. clay dispersal by organic acids or weathering of rock substrate) |
Loss of tensile strength |
Loss of tensile strength by dieback of vegetation, e.g. from burning, air pollution or in extreme cases of grazing and/or poaching |
Increase in buoyancy |
Increase in buoyancy through formation of subsurface water pools (e.g. to form quaking bog) or filling and/or blocking of natural pipe networks (e.g. through pipe ceiling collapse or excess rainfall) |
Table 2.3. Triggers for peat instability
Natural triggers
Trigger |
Influence on stability |
Restoration impacts |
---|---|---|
Intense rainfall |
Causes development of transient high pore-water pressures along pre-existing or potential rupture surfaces (e.g. at the discontinuity between peat and substrate) |
n/a |
Snow melt |
Causes development of high pore-water pressures |
n/a |
Rapid ground accelerations (earthquakes) |
Causes a decrease in shear strength |
n/a |
Unloading by fluvial incision |
Reduces support to upslope material |
n/a |
Artificial triggers
Trigger |
Influence on stability |
Restoration impacts |
---|---|---|
Alteration to drainage patterns |
Focusses drainage and generates high pore-water pressures along pre-existing or potential rupture surfaces (e.g. at the discontinuity between peat and substrate) |
Blocking of pipes by drain reprofiling / damming |
Rapid ground accelerations (blasting / vehicles) |
Causes an increase in shear stresses |
Plant operations during restoration works |
Unloading by excavations |
Reduces support to the upslope material (e.g. during track construction) |
Reprofiling activities on transverse drains |
Loading by plant or overburden |
Causes an increase in shear stress |
Plant operations during restoration works (failure to use low ground pressure excavators) |
Again, when considering the potential impacts of restoration, it would primarily be the effects of short-term groundworks associated with restoration that might act as triggers.
2.2.5. Consequences
Peat landslides are usually only identified when they have consequences, and investigations into how they might have been prevented or their consequences minimised are typically undertaken only where human activities have contributed to their occurrence.
Good practice guidance (e.g. Scottish Government, 2017) has been driven by well publicised events, such as the Derrybrien bog burst in Co. Galway. This failure occurred in October 2003 in association with groundworks for the Derrybrien Wind Farm, inundating local watercourses and causing significant fish kill (Lindsay and Bragg, 2005). Naturally occurring peat slides and peaty-debris slides occurred on Dooncarton Mountain (Co. Mayo; Creighton and Verbruggen, 2003) and in Channerwick (Shetland, Mills et al., 2007) on 19th September 2003, both impacting local roads, with the former surrounding houses and washing out a graveyard and the latter polluting local watercourses and affecting marine shellfish (Henderson, 2005). In December 2016, a plant operator was killed during excavation works in peat at the Derrysallagh wind farm site in Co. Leitrim (Flaherty, 2016) on a plateau in which several published examples of instability had been previously reported.
Table 2.4 provides a list of at-site and off-site impacts that may arise from peat landslide events.
Given that a key aim of restoration is to improve habitats and protect the natural carbon sinks that peatlands represent, negative environmental impacts through loss of peat and reduced long term carbon storage must be avoided by appropriate selection of restoration sites and restoration groundworks.
The magnitude of the consequence is dependent on the type and size of failure and its connectivity to other sensitive receptors. For example, bog bursts typically evacuate less of their source areas than peat slides, and therefore total peat and embedded carbon losses may be less, however, their runout may be more fluid and disruptive to connected watercourses. Consequences are best considered within a risk framework in association with likelihood – this is described as an introduction to the risk protocols in Section 5.1.
Table 2.4. Potential impacts from peat landslide events (after Warburton, 2015)
Impact location |
Short term |
Long term |
---|---|---|
At landslide site |
|
|
Off site (downslope or downstream) |
|
|
2.3. An overview of peatland restoration
2.3.1. Background to peat restoration
Peatlands are now recognised as globally important providers of ecosystem services, including for provision of food, water supply, climate regulation, biodiversity, recreation and cultural heritage (Lantschner et al., 2011; Bonn et al., 2016). In their degraded state, they may pose a threat to health in the event of peatland fires and contribute significantly to carbon dioxide emissions (Page et al., 2002). Although peatlands cover less than 3% of the world’s surface, it is estimated that they store double the amount of carbon held in global forest biomass and more than 30% of the total global soil carbon store (Bonn et al., 2016). Peatlands make up some 9.5% of the UK’s land area with an estimated carbon store of over 3,200 million tonnes, of which 1,600 million tonnes are estimated to be in Scotland (Bonn et al., 2016).
The significance and importance of UK peatlands is evident in their protection by a range of legislation and national initiatives including the UK Biodiversity Action Plan, the EC Habitats Directive, the Water Framework Directive and the Climate Change Act (Lantschner et al., 2011). In Scotland, where much of this resource is located, there is a considerable body of legislation and guidance supporting land use and land management in peatlands, including: the National Peatland Plan (SNH, 2015a), Wind Farm Developments on Peatlands (Scottish Government, 2011), Regulatory Position Statement – Developments on Peat (SEPA, 2010), Deciding Future Management Options for Afforested Deep Peatland (FCS, 2015), the Scottish Land Use Strategy (Scottish Government, 2016), the Scottish Government's discussion paper on the Management of Carbon-Rich Soils and the Scottish Soils Framework (Scottish Government, 2009).
Evans and Warburton (2007) note that blanket peatlands in the UK and Ireland are distinct by the degree and intensity of physical erosion to which they have been subject over the last 1,000 years. Where peatland degradation is severe, erosion and weathering can change peatlands from carbon stores to carbon sources. Conversely, where peat continues to accumulate, it may continue to act as a carbon sink. Alderson (2019) notes a long history of development of restoration approaches in the UK, typically aiming to restore hydrological function, vegetation cover and active peat forming vegetation. Restoration success is typically measured by monitoring of water tables (for hydrology) and vegetation surveys (for cover and composition).
2.3.2. Peatland ACTION
Peatland ACTION was established in 2012 to support restoration of damaged peatlands in Scotland, with over 19,000ha benefitting from groundworks to restore hydrology and vegetation. Funding for restoration of both publicly and privately owned peatlands is provided by the Scottish Government, with independent site appraisal and technical advice offered by a team of Peatland ACTION Project Officers within NS and partner organisations. Applications for funding are made on an annual funding cycle and require characterisation of peat depth and peatland condition across the proposed restoration area using a series of guidance notes. Submission of applications supports ongoing assessment of peatland condition within Scotland while the growing list of completed projects provides an evidence base against which to judge effectiveness of the initiative.
The next section provides a brief overview of typical restoration settings and techniques, leading onto consideration of specific impacts of these techniques with respect to peat stability in Chapter 3.
2.3.3. Restoration in different peatland settings
In addition to the condition of the peatland, the type of groundworks associated with restoration may vary according to the setting being restored: blanket bog or raised bog. A brief summary of these different settings is provided below.
2.3.3.1. Blanket bog
Evans and Warburton (2007) describe a range of hydrological and topographical mire settings into which peatlands can be subdivided. Although there are other criteria with which to classify peatlands (e.g. based on habitat), it is hydrology and topography that typically control geomorphological activity and in turn slope stability. Raised mire (or bog) and blanket bog are the two dominant hydro-topographical settings considered within Peatland ACTION funding applications, and conceptual models are shown in Figure 2.11. Additional settings include basin mires, valley mires, floodplain mires and sloping mires, which, where they are linked across a landscape, can be referred to as blanket mire complexes (Lindsay, 1995).
Blanket bogs are ombotrophic systems, deriving their moisture and nutrients from rainfall. In contrast with peatlands elsewhere in the world, peatlands of the UK and Ireland are extensively eroded (Evans and Warburton, 2007). Gullying and sheet erosion are common features of UK and Irish bogs and peat instability is most commonly documented. Common geomorphological features of blanket bogs are shown on Figure 2.12. Instability features are shown on Figure 2.6.
In Scotland, blanket bog tends to either completely conceal the underlying hard rock substrate (i.e. a true ‘blanket’) or in areas of rugged geology, fills the hollows between areas of upstanding rock outcrop to form a discontinuous cover of highly variable peat depth. Review of peat landslide locations indicates that peat instability is overwhelmingly reported in the former setting rather than in areas of rock outcrop.
Blanket bogs are subjected to a range of human activities which may act to degrade the peat or alter its hydrology or geomorphological activity:
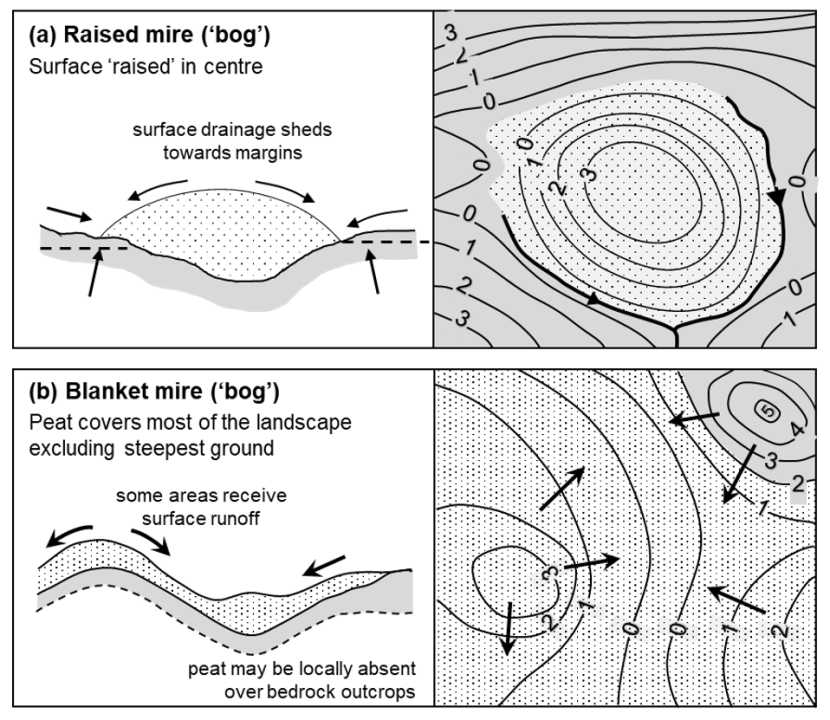
Raised bog hydro-topographical model showing a typically domed profile, with water shedding towards the margins and (b) blanket bog hydro-topographical model showing peat covering the underlying topography other than the very steepest slopes. Drainage directions vary with underlying topography.
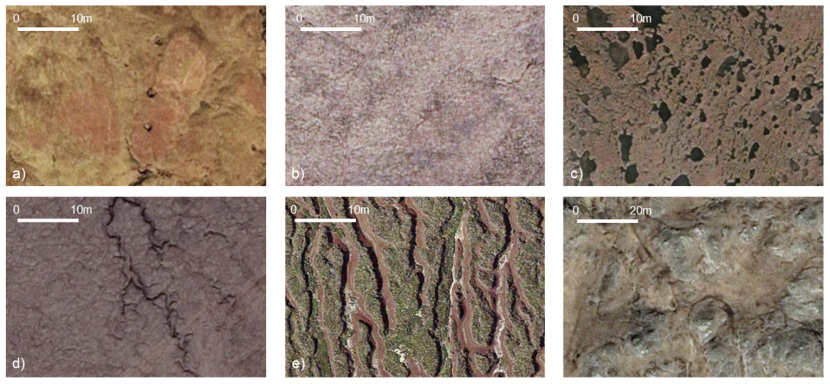
Aerial images of common geomorphological features of blanket bogs, a) planar with pipes, b) planar (no other features), c) planar (with pools), d) slightly eroded (isolated gullies), e) heavily eroded (extensive hagging), f) undulating peat among rock outcrops.
- Afforestation: viewed as poor quality soils, large areas of upland and lowland peatland have been afforested since the 1970s, typically with conifers, which can be commercially viable on shallower peats. Ground disturbance in preparation for planting can be considerable, with significant damage to the acrotelm through ploughing of closely spaced ridge and furrow, and compartmentalisation of the catotelm through cutting of collector drains, often to substrate. The water table typically falls to the height of the furrow base, drying both the ridges (Sloan et al., 2018) and furrows (Lindsay and Bragg, 2005), while desiccation cracking may occur with irreversible drying of the upper peat layers as the growing crop draws water from the peat soil (Lindsay and Bragg, 2005). Hobbs (1986) notes that post-felling, there may be a rebound effect from the removal of the weight of trees, which have their root plates within the peat.
- Drainage: extensive post-war drainage of peatlands in the UK and Ireland took place in order to help make uplands more productive for agriculture. The purpose of drainage was to lower water tables, remove surface water and improve vegetation for grazing and game (Holden et al., 2017). An estimated 1.5 million hectares of blanket peatland have been drained (Stewart and Lance, 1983). Drainage densities, patterns and drain orientations (e.g. along contour, oblique or directly downslope) vary considerably within and between locations. In all cases, drains act to draw down the water table near to the drain and compartmentalise the peat. Holden (2005) suggests that pipe frequency is strongly controlled by drainage, being more prevalent in areas of artificial drainage, which, given the suspected role of pipes in contributing to peat instability (Warburton et al., 2004), is significant.
- Cutting: cutting of peat for fuel in areas of common land is a long-established practice in upland peatlands of the UK and Ireland, and is known as turbary. Cutting involves the removal of the surface mat of vegetation (i.e. the acrotelm) known as ‘flaying’, and then cutting of bricks of peat from a vertical peat face. The surface mat is then replaced in the cutting floor. Typically, the bare face dries over time, though the penetration of drying into the peat behind the face is limited as the hydraulic conductivity of the peat face is reduced as it dries (Lindsay and Bragg, 2005). The primary effects of cutting on stability are where the toe of a slope is progressively removed by cutting or in larger areas of industrial cutting where tines (slots) are cut to substrate using Difco machines to extract peat. These tines have been implicated in failure at both Pollatomish and Tullynascreen (Dykes and Warburton, 2008).
- Burning: prescribed burning as a land management tool (‘muirburn’) is widely used across Scotland to control vegetation cover, limiting growth of shrubs and trees and encouraging heather and grassland. Wildfires may also significantly affect peatlands, being uncontrolled and potentially burning for longer periods. Chapman et al. (2017) found there was limited evidence in relation to the effects of burning on the hydrology of peat, with some suggestions that unburnt peat had a lower water table than burnt peat due to higher water uptake by deeper rooted vegetation in unburnt areas. Feehan and O’Donovan (1996) suggested that peat burning may reduce the stability of peat.
The impacts of these activities are typically the focus of restoration work, which looks to address lowering of water tables in drained peatlands and return an undulating macrotopography to a more level peat surface to support more even distribution of surface water, arrest drying and encourage recolonisation by peat forming vegetation. Specific restoration techniques and their impacts are described in Section 4.
2.3.3.2. Raised bog
Like blanket bogs, lowland raised bogs are ombotrophic and are distinct from blanket peat by their typically domed morphology, in which peat is at its deepest in the centre (sometimes up to 10m). In their lowland settings, they are often surrounded by forestry or agricultural land (Lindsay and Immirzi, 1996).
In comparison with blanket bogs, raised bogs are relatively isolated and discrete with well-defined margins. In an inventory of UK raised bogs by Lindsay and Immirzi (1996), at least 800 individual raised bog units were identified within Scotland, with the majority (c. 85%) being less than 60ha in area.
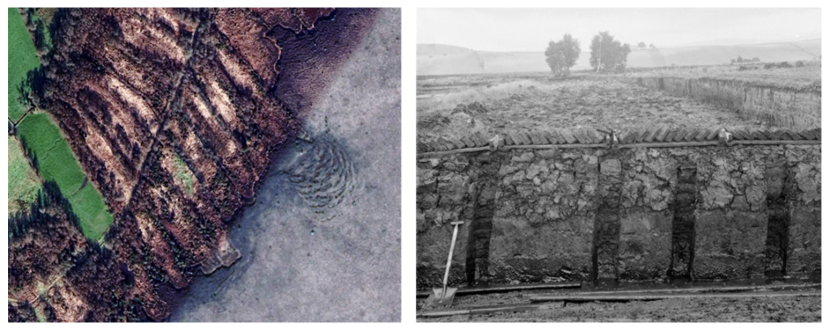
Structural damage to peat associated with cutting and drainage – removal of toe support at Carn Park raised bog (left) and section view of drying of upper peat (Black Moss of Dinnet; McIntosh, 2020).
Given their relatively small size and occurrence on minimal gradients or flat ground, they usually have significantly fewer geomorphological features than blanket bogs, with occasional gullying and erosion at their margins (sometimes reaching towards their centres).
In common with blanket bogs, raised bogs have been exploited for their peat soils as fuel and have been drained and planted for commercial forestry. Many of the impacts of these activities are similar to those described for blanket bogs and are not repeated here. Lindsay and Immirzi note that cutting can result in strong changes in hydraulic gradient within raised bogs with water table drawdown and loss of hydrological function of the acrotelm. An example of damage to raised bog structure associated with cutting is shown in Figure 2.13.
In addition, the lowland setting of raised bogs and their proximity to urban areas has led to significant land reclamation, with many of the raised bog footprints significantly smaller than they would have been in their natural state.
Restoration activities in lowland raised bogs have similar aims to those for blanket bogs, though the discrete nature, gentle topography and minimal slopes of raised bogs enables additional techniques such as bunding to be adopted to retain surface water. Again, the impacts of these techniques and their implications for stability are addressed in Section 4.
3. Determining the potential effects of restoration techniques on peat stability
3.1. Desk-based review of restored Peatland ACTION sites
3.1.1. Approach
In order to evaluate the effects of restoration activities on the stability of restored sites, a spatial database of Peatland ACTION project locations was reviewed against publicly available satellite imagery in Google EarthTM. The objectives were as follows:
- To determine whether satellite data are of sufficient resolution to identify restoration work.
- To determine whether restored sites show any signs of accelerated geomorphological processes (such as erosion), pre-failure indicators (such as cracking or ground displacement) or peat landslides.
- To provide evidence-based context with which to evaluate the potential for restoration to precondition or trigger peat landslides.
Sites considered comprised all Peatland ACTION projects since inception of the initiative in 2012 up to May 2019. While at least one epoch of high resolution satellite imagery was available across Scotland, the year of acquisition varied across the country. Use of the historical imagery function in Google Earth ProTM enabled identification of the date of imagery and comparison with the funding and delivery year of restoration. The Peatland ACTION database included centre points for each project, the name of the project and the project status (e.g. ‘Completed’, ‘Deferred’; Figure 3.1).
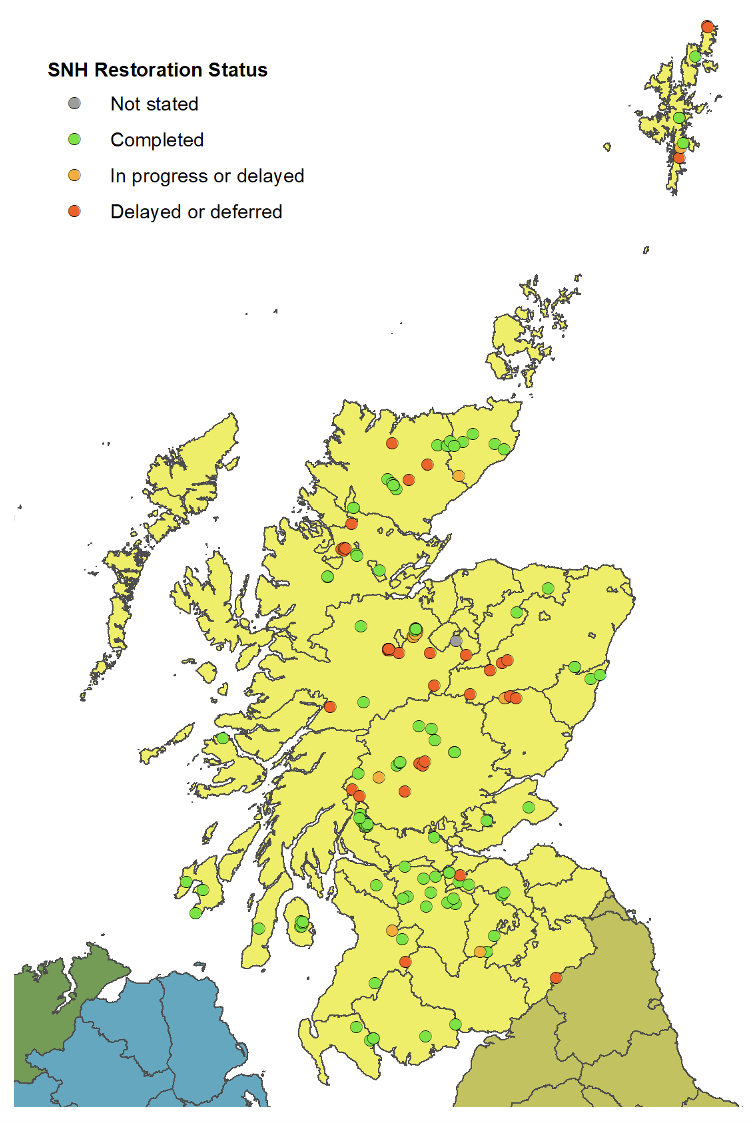
Status of restoration locations (complete/ delayed / deferred) in Peatland ACTION spatial database, up until end 2019.
3.1.2. Results
Of the range of restoration techniques typically employed within upland and lowland peatlands, only a small number are observable on satellite imagery of the resolutions available up to 2019. These were:
- Ditch blocking, though the mode of blocking was not usually clear.
- Ditch reprofiling.
- Gully blocking.
- Felling and/or ground smoothing.
- Surface bunding.
Imagery corresponding to each of these techniques is shown in Figure 3.2. Satellite imagery was available for numerous epochs between 31/12/2004 and 26/08/2019. In most cases, resolution was comparable across multiple epochs between different locations, although time of year for acquisition meant that snow cover sometimes impeded view of the ground, or cloud cover obscured ground features. In many cases, the epoch of data acquisition preceded the timing of restoration work, meaning no conclusions could be drawn.
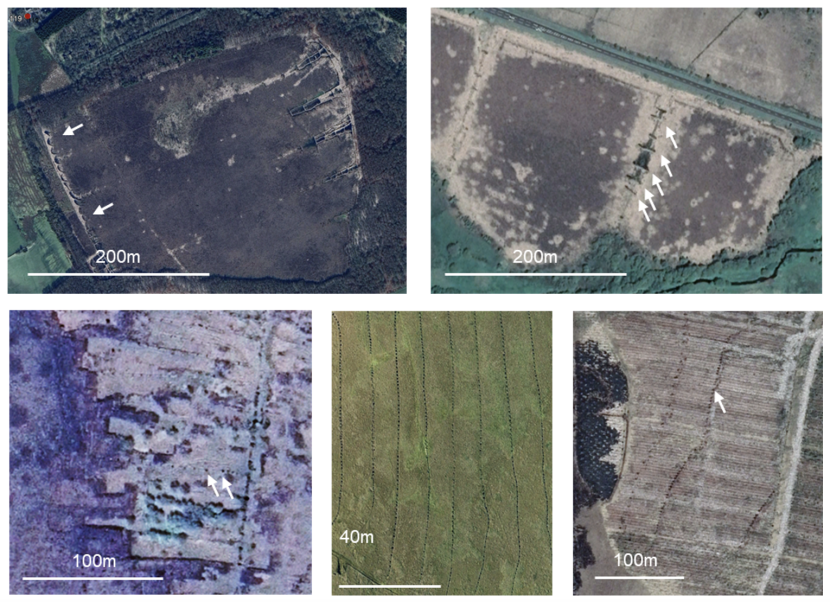
Restoration groundworks visible on satellite imagery, clockwise from top left: surface bunding at Carsegowan Moss, Peat and composite dams at Dalmellington Moss, furrow blocking at Dyke Plantation (visible as lines to the right of peat dams), high frequency peat and pile dams on open blanket bog at Forsinard (visible as dots on each drain), blocking of drains at cuttings in raised bog (Moss of Crombie).
The 568 individual locations reviewed comprised 206 areas within which restoration had been planned or undertaken. Many areas comprised multiple phases of restoration (typically 2 or 3), with examples including restoration of gentle parts of a site followed by restoration of steeper slopes (e.g. at Ben Lomond).
Of these 206 areas, 100 areas had satellite photography available after the proposed restoration period. Of these:
- 41 were either stated to be raised bog or were interpreted to be raised bog (from satellite imagery), and 59 were blanket bog.
- 35 were commercially or fully afforested, 32 part-afforested or informally forested and 33 non-afforested prior to restoration.
- 87 were drained (either as part of forest works or in non-afforested areas) and 13 were undrained.
- 12 were eroded (typically gullied or comprising isolated peat haggs or eroding blanket peat margins) and 88 uneroded.
Inspection of each site at maximum resolution (typically an “eye altitude” of c. 1km) indicated the vast majority to show no signs of instability, landslides, drain offsets, tension cracks / tearing, enhanced erosion of gullies or drains, excessive ponding or waterlogging.
Exceptions were:
- A shallow landslide present between blocked drains in non-afforested blanket peatland (Caithness): inspection of historical satellite imagery indicated the failure to have taken place prior to drain blocking and therefore restoration was not likely to have been a contributory factor.
- Margin failure of a restored lowland raised bog at Moss Band (Lanarkshire): this location was observed to have failed subsequent to restoration and was one of the events contributing to the request of the current study.
- Margin failure of a restored lowland raised bog at Greenhead Moss (Lanarkshire): this failure occurred subsequent to restoration and also contributed to the request for this study.
All three sites were visited during fieldwork undertaken for this study and are considered in Section 3.2 alongside other case study sites.
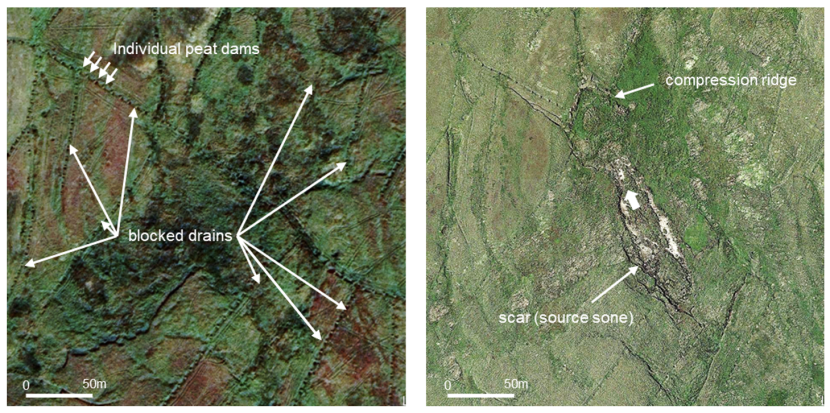
As part of the peat landslide database review, one example of instability was identified in association with drain blocking, but outside Scotland (see Figure 3.3). The landslide occurred at Coverdale in Yorkshire, and was one of five failures to occur during a single rainfall event. The other four failures did not occur in areas of drain blocking. Review of pre-failure and post-failure satellite imagery indicates that the drain blocking had occurred prior to the landslide, though it is not clear whether there was any influence from drain blocking (particularly given the occurrence of the other slides).
3.1.3. Conclusion
The availability of a spatial database of restoration projects is valuable in monitoring ongoing peatland responses to restoration groundworks. While widespread restoration is a relatively recent activity and full satellite coverage of Scotland has only recently been achieved, there is now sufficient information available to remotely evaluate the success of restoration as evidenced in ground cover changes and softening of drain and gully morphology (for example).
In relation to this study, it is clear that although restoration works have been carried out in a wide range of settings, from lowland raised bogs to hillslopes in blanket bog, ground instability is rarely observed, certainly in comparison to groundworks in other settings (such as quarrying, track or wind farm construction, see Section 2.2.4). This said, the period of review is short (c. 10 years since Peatland ACTION’s inception), and hydrological, ecological and geomorphological changes in response to restoration may occur over a much longer time period (e.g. Holden, 2005).
As time continues to pass for these existing projects and as new restoration projects are being funded, the evidence base for assessing the effects of restoration (on drainage, habitat and instability) will continue to grow and provide confidence in the value and effectiveness of the Peatland ACTION initiative.
3.2. Field-based review (case studies)
In order to understand the potential effects of restoration activities on ground stability, case study sites were visited with Peatland ACTION officers to inspect areas of past instability. Other sites with known instability for which restoration is proposed were also inspected. These case study sites were in a range of settings and are as follows:
- Case Study I: margin failure of restored Mossband lowland raised bog
- Case Study II: margin failure of restored Greenhead Moss lowland raised bog
- Case Study III: gully head / drain failure in upland blanket bog proposed for restoration
- Case Study IV: failure in drained upland blanket bog
- Case Study V: forest to bog restoration (no instability at this site)
These site visits enabled observation of a range of restoration groundworks, including drain reprofiling, drain blocking using peat and composite dams, surface bunding and trench bunding.
In addition, a number of restoration sites were visited with Forestry and Land Scotland (Case Study V) in order to review a range of forest-to-bog restoration groundworks including stump flipping, cross-tracking and ground smoothing.
Key findings are summarised below.
3.2.1. Case Study I - margin failure of restored lowland raised bog (Mossband)
Mossband is an afforested lowland raised bog that has recently undergone restoration groundworks, located immediately to the west of Shotts in North Lanarkshire. An assessment of the restoration potential of the forest by the Scottish Lowlands Forest District (2014) indicated Mossband to comprise a typical domed lowland raised bog (LRB) of deep peat. This assessment noted that the bog had been drained and prepared for planting, the latter taking place between 1981 and 1990, although the central dome was not planted. Peat depths average 3.0-4.0m in the area near the failure. Drain alignments in the unplanted area are clear on satellite imagery and date from the 1960s/1970s (FLS, 2019). Pre- and post-restoration imagery is shown in Figure 3.4.
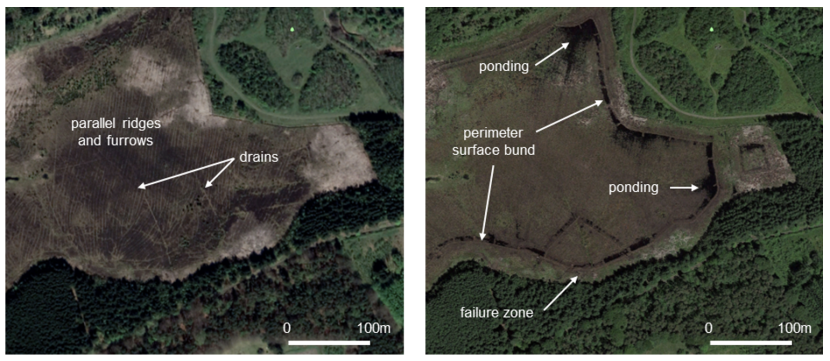
Restoration groundworks included drain blocking, removal of natural regeneration by pulling / cutting, ground smoothing and installation of a trenched peat bund around the perimeter of the bog. In winter 2018/2019, a small area on the south side of the bog slid a few metres south towards the treeline, stalling against it (Figure 3.5).
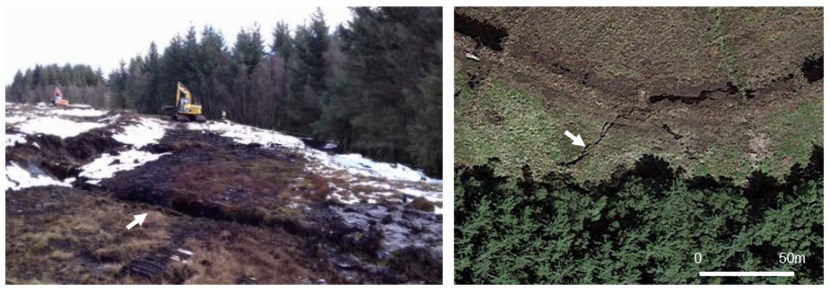
A site visit was undertaken in September 2019 by EPG accompanied by the Peatland ACTION officer who had overseen the funding application for restoration.
Findings were as follows:
- Ground smoothing (to remove the ridge and furrows) had been very effective, with little surface evidence of ridge and furrow visible on the ground (or on satellite imagery). Substantial revegetation had taken place.
- The trench bund had been cut to around 1.0m below the ground surface and was elevated above it by 0.3m on average.
- The aim of the bund was to limit surface and near surface overland flow beyond the perimeter of the bog.
- A number of key drainage pathways on the bog surface (both natural low points and artificial drainage lines) were blocked using composite dams.
- The effectiveness of both damming and the perimeter trench bund were visible in pooling of water against the bund around the site (see Figure 3.5).
- In some locations, trampling of the bund (either by stock or by the public) had caused lowering, such that continued trampling or increase in ponding might overtop and erode the bund, releasing water and entrained peat over the margin of the bog.
- Some composite dams showed limited evidence of bowing, allowing small quantities of slurried peat to escape through gaps in their structures.
- The failure zone comprised a wedge-shaped area in plan, translated outwards towards the bog margin by a few metres leaving a wide and deep tear in the peat behind it along which water from the raised bog continued to drain. A small compression ridge was visible at the toe (downslope limit) of the wedge.
- The wedge failure did not have characteristic peat landslide morphology given its significant peat depth, with a single intact raft, minimal subsidence and very minor outflow of material below the failure zone.
The Peatland ACTION officer noted that the zone of failure was at a low point in the raised bog towards which natural drainage focused. Subsequent to the failure, remedial works were undertaken to limit further instability and repair damage to the bund caused by the landslide. These included construction of a new inverted v-shape bund behind the headwall of the slide and diversion of drainage to the east of the slide mass. There has been no subsequent movement at the slide location, although it remains waterlogged.
Additional interpretation of the site was undertaken subsequent to the site visit using satellite imagery, historical maps and a 5m DTM processed to remove tree cover. Findings were as follows:
- Historical maps indicated Mossband Wood to have been fully tree covered in the late 1800s with a small quarry in the centre north of the site visible by 1899. An old shaft is noted east of the failure location on the 1921 map.
- Much of the tree cover had been felled by 1957, and the southern boundary of the bog was bounded by a track running east to west. The quarry no longer appeared to be worked with the access track to it no longer shown.
- Drains cutting to the south and joining a peripheral drain are visible on the 1957-1958 map. One of these drains feeds the failure zone. Subsequent maps show no significant changes.
- Comparison of pre-restoration imagery with the digital terrain model indicates the peripheral drains and two drains to the south to be crosscut by extensive ridge and furrow (which would act to channel surface water towards the south and west) where elevations are at their lowest.
- A transect from north-to-south across the bog and using interpolated peat depths between sample points derived from the original grid survey shows the basal surface of the bog to dip to the south beneath the raised bog. Review of 0.25m elevation contours indicates that the slope of the raised bog margin is steepest in this area, and that surface topography also focuses drainage to the south (see Figure 3.6).
Taken together, failure would appear to have occurred at this location because:
- The margin of the raised bog is steepest at this location.
- The contact between peat and substrate dips towards the location.
- Drainage is focused in this location both in the catotelm (due to the dip of the contact between peat and substrate) and in the acrotelm (bog surface).
- Historically, the toe of the slope may have been cut to construct the track, oversteepening the margin of the raised bog.
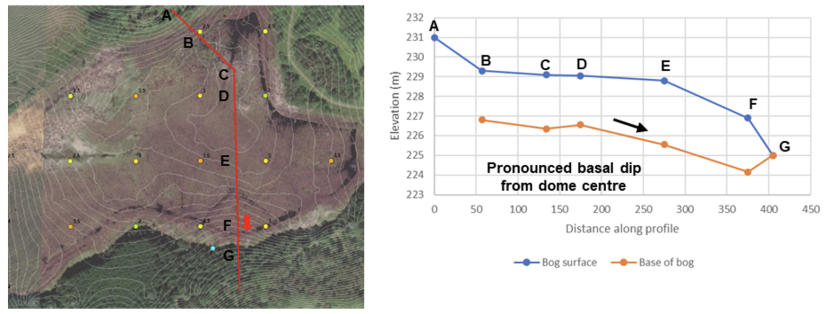
Image (left) showing surface topography (0.25m contours), failure location (red arrow), peat depths (coloured dots) and indicative bog surface and basal profiles for Mossband. The peat mass is located between the ‘bog surface’ and the ‘base of bog’ on the profile on the right.
3.2.2. Case Study II - margin failure of restored lowland raised bog (Greenhead Moss)
A second lowland raised bog within North Lanarkshire, Greenhead Moss near Wishaw, also experienced instability after restoration. The site comprises a typical domed bog that had been extensively cut between the 1930s and 1980s. In the present day, the main path through the site lies above the lowered peat surface. A series of hollows (from which peat was cut) and baulks (residual raised areas between hollows that are now drying) make up much of the footprint of the bog. In between the baulks and hollows are drains roughly 1m wide which drain from the northeast to southwest (Peatland ACTION, 2015). Peat depths are reported as between 2.0m and 4.7m based on around 16 samples distributed across the site. Quarrying to the west of the site subsequent to 1945 left a steeply sloping engineered slope, and it is in this location that the margin of the bog collapsed in December 2015 subsequent to heavy rainfall. Pre- and post-restoration imagery are shown on Figure 3.7. Pre-quarrying imagery from 1945 is shown in the left image.
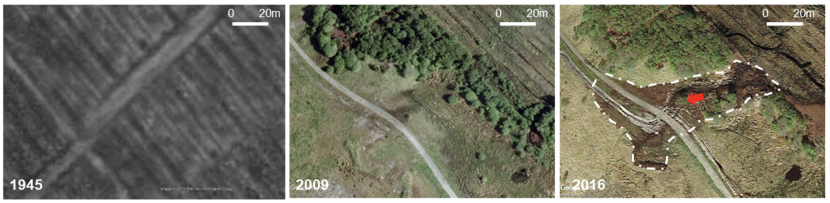
Initial restoration groundworks were completed by March 2015 and comprised:
- Reprofiling of baulks to prevent them acting as barriers to water movement.
- Blocking of the drains adjacent to the banks using cut tree regeneration and peat from the banks in order to slow water flow and raise water tables.
- Installation of plastic dams within the drains.
- Relocation of peat to both the northwest and southwest of the bog to block a large drain in the former and form a surface bund for water retention in both locations.
In December 2015, following sustained heavy rainfall (Peatland ACTION, 2016), an area of the southwest margin failed and overran the public footpath running to the west of the bog. Subsequent remedial works were completed by early March 2016, including installation of a trench bund to seal the previous location (which had been actively eroding and discharging water since the initial failure), installation of pipe culverts under the footpath and piling dams to compartmentalise the site and direct water away from the failure location. Shortly afterwards, a second phase of movement occurred alongside further seepage and erosion. Deep subsurface cracks were observed to be allowing water to build up against the baulks to bypass the bund.
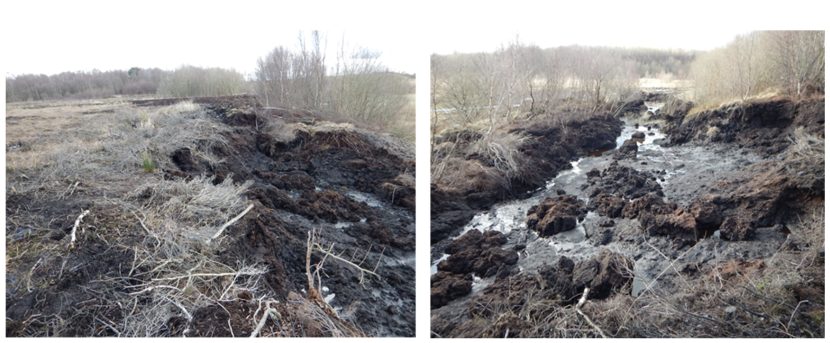
The site was visited with the Peatland ACTION case officer in September 2019. Findings were as follows:
- The trench bund that post-dated the first failure but preceded its reactivation had been cut within 2m of the bog margin, and this may have contributed to the collapse by disrupting any tensile strength remaining in the acrotelm.
- The peat was particularly deep at the margin and continued to discharge water from its headscarp indicating active water movement towards this location.
- There was little evidence of further retrogression (cut back) behind the scarp.
A desk study review of the site was undertaken using 5m DTM data, historical map data and peat depth data. As with Mossband, the base of the raised bog dips towards the area of failure, as does the surface topography (Figure 3.8).
Review of a series of satellite images at the failure location indicates that the engineered slope retaining the bog (which would have been continuous to the southwest prior to quarrying, see Figure 3.7) may have had the smallest footprint at this location, with the least tree cover. Therefore, this point on the engineered slope may have acted as both a low point and a pinch point for retention of the bog peat, the margins of which had previously been fully cut away by peat workings post 1945.
In summary:
- The initial phase of failure is likely to have occurred as a function of concentration of drainage in the southwest margin of the bog, in part caused by the dip of the base of the bog towards the southwest.
- The second phase of failure may have been encouraged by trench bunding into the acrotelm in proximity (c. 2m) to the headwall of the initial failure, disrupting the remaining tensile strength within the upper metre (acrotelm of the bog).
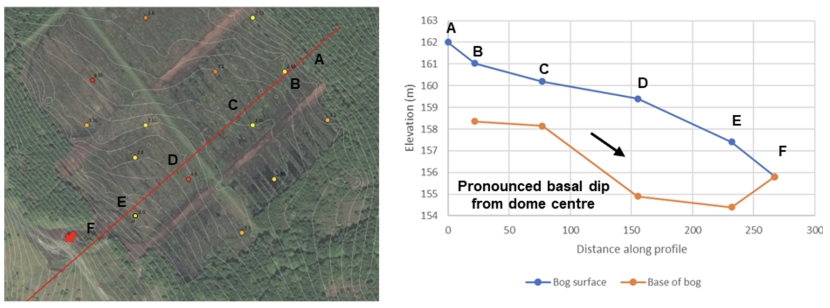
Image on the left showing surface topography (0.25m contours), failure location (red arrow), peat depths (coloured dots) on Greenhead Moss. Indicative bog surface and basal profiles (the peat mass is located between the ‘bog surface’ and the ‘base of bog’) on the profile on the right.
In contrast with Mossband, extensive reworking of the top surface of the bog had taken place through reprofiling of the baulks and hollows, and therefore it is likely that much of the upper structure of the peat had been disturbed by past cutting activity.
3.2.3. Case Study III - gully head / drain failure in upland blanket bog proposed for restoration (Cairngorms)
A third site was visited in the Tulchan Estate in the Cairngorms, within which previous successful drain reprofiling had been undertaken and for which further reprofiling has been specified. The site is an extensively drained blanket bog in the north of the Cairngorms and has experienced small scale slope instability at gully heads into which the drains discharge (Figure 3.10). One of these failures occurred subsequent to drain reprofiling and was reviewed as part of the site visit.
Restoration works were reviewed in the field with the relevant Peatland ACTION officer and comprise a combination of drain reprofiling and dam installation. Many of the drains were along contour or oblique to contour, and intercepted downslope pipe networks, visible by collapsed pipe ceilings at the bog surface. Where pipe alignments were traceable at the surface, ponding was visible within the cross-cutting and now dammed drains. There was no evidence of instability of the drains, either in the form of offsets or bulging. Peat depths were typically 1.0-2.0m, but locally deeper. Examples are shown on Figure 3.11.
Review of the failure location indicated that the surface of the feature appeared to be well below the base of the peat, and it is likely that post-failure erosion had cut into the substrate rather than that this mineral material was incorporated in the initial failure. The majority of landslide runout from the initial failure appeared to be mineral in nature, as can be seen on the left panel of Figure 3.12). The stream present at the toe of the failure did not seem to have been displaced by landslide material and did not appear to have cut the toe of the slope to trigger failure.
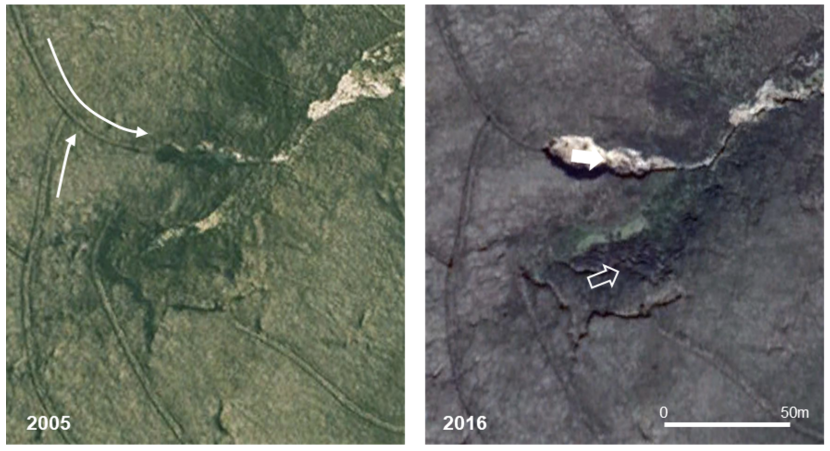
Tulchan Estate drainage and peaty-debris slides, images from 2005 (left) and 2016 (right). The solid arrow on the 2016 image shows most recent phase of failure, whist the earlier footprint can be seen on 2005 image. The hollow line shows older failure; mineral nature of runout visible as light textures in top right of each image.
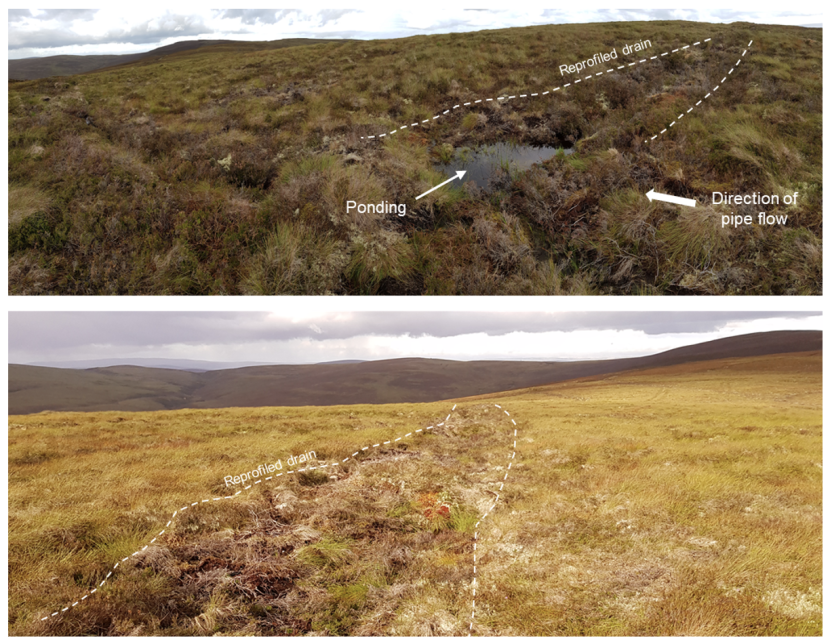
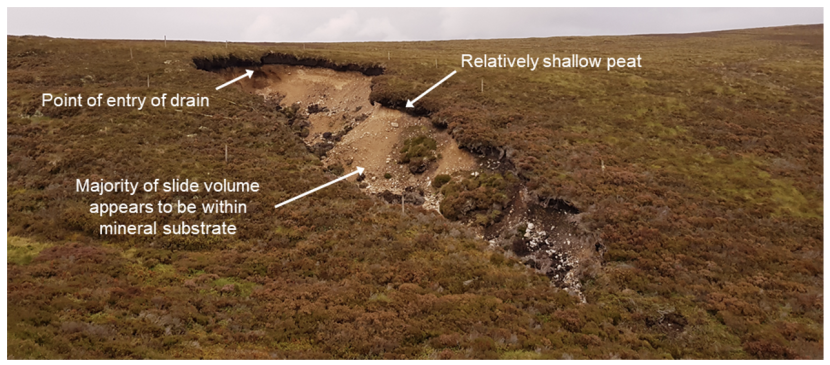
Adjacent to the failure, drain reprofiling had been undertaken to within a metre of significant tears in the peat (indicating marginal stability, the arrowed location on Figure 3.10, and Figure 3.13).
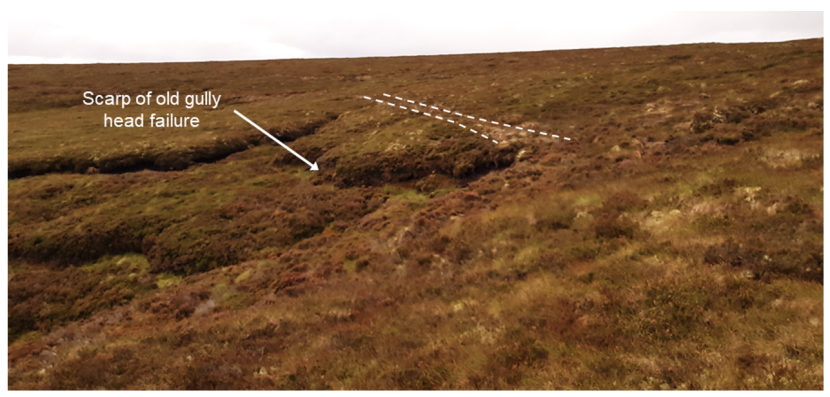
3.2.4. Case Study IV - failure in drained upland blanket bog (Caithness)
The fourth site was located within the Welbeck Estate, Caithness, and comprised a peat slide in a drained area of blanket bog. Drain blocking work had been undertaken elsewhere within the Estate including within the vicinity of a second peat slide positioned between two contour aligned drains. Both features are shown on Figure 3.14. Review of historical satellite imagery indicated that both peat slides occurred prior to 2004 and therefore drain blocking is not implicated in either failure. Although the latter failure could not be visited due to access restrictions relating to stalking, the former feature was subject to a site visit accompanied by two Peatland ACTION officers.
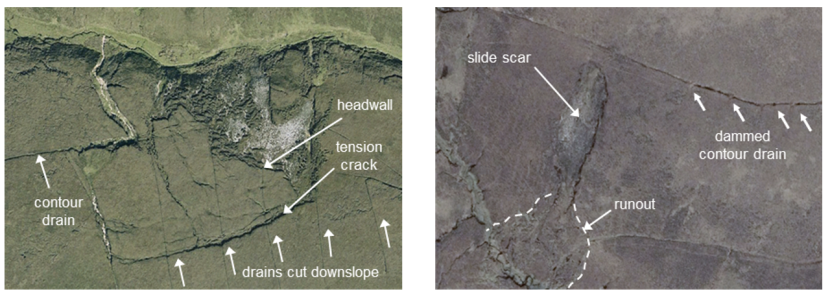
Aerial images of two peat slides within the Welbeck estate, showing the orientation of nearby drainage ditches.
Review of the landslide on the ground indicated it to be a good example of a peat slide with characteristic diagnostic features including large-scale rafting, thrusting at the margins of the slide, a discrete headwall and blocky runout (Figure 3.15). Pipes were visible in the headwall of the main slide. These pipes are likely to have existed at the time of failure since they would not have formed once the large tension crack developed further upslope. The exposed shear surface was well vegetated with rushes and grasses indicating it to be at least 10-20 years old. Peat depths were typically 1.0m-1.5m around the margin, with basal peat being of von Post scale H7-H8.
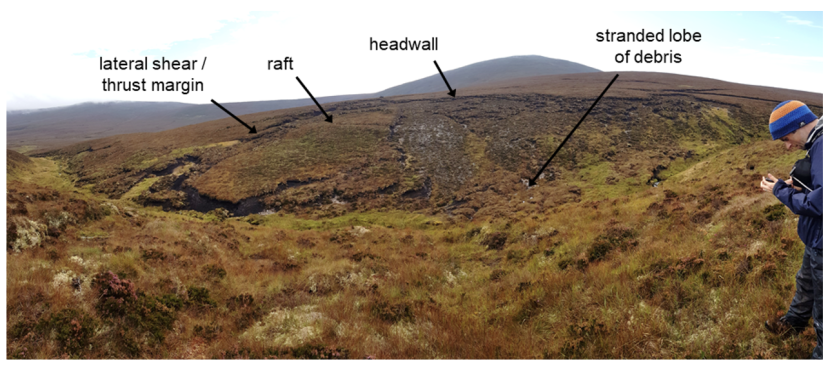
Image showing peat slide visited as part of the site visit to Welbeck estate, annotated to show the headwall of the failure, lateral shear zone, and rafted debris.
The toe of the slide was directly coupled with a steep sided watercourse, with the main body of the slide straddling the convexity separating the valley side from the more gentle planar slopes above. The rafting of material over the east lateral shear indicates that the upper slope may have displaced first, loading the lower slope and causing its subsequent failure into the stream. Debris below the slide appeared to stop short of the stream which had not shifted from its original course (and therefore was unlikely to have triggered the slide at the toe).
In summary, it is possible that the contour aligned drains present at the site were involved in the failure, possibly becoming blocked through minor slippage and then allowing build-up of water behind the headscarp of the uppermost raft, ultimately triggering failure.
3.2.5. Case Study V - forest to bog restoration at Forestry and Land Scotland research site (near Lairg)
The final case study site comprised forest-to-bog restoration works within a Forest and Land Scotland (FLS) research site. The site visit by EPG was accompanied by an experienced peatland asset forester from FLS. The primary purpose of the site visit was to observe ground smoothing using stump flipping and cross-tracking in an area of deep peat, a relatively new technique which aims to produce a very low relief surface with minimal macro-topography, encouraging revegetation by peat forming species.
The site was being worked by a 21t low ground pressure excavator running on 1500mm tracks (Figure 3.16). The plant operator noted that higher ground pressure excavators were required for ground smoothing and stump flipping work to provide sufficient counter to the resistance from the brash and wood when driving them into the peat, as well as to make cross-tracking effective.
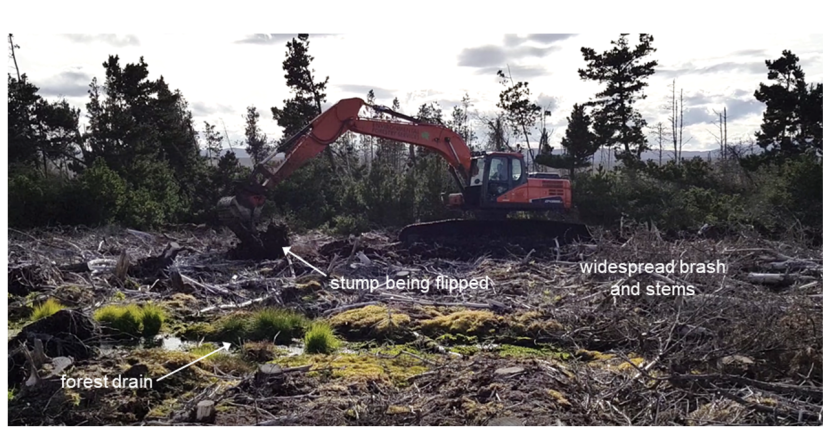
Large areas of windthrown trunks, brash and stumps were turned using the excavator bucket, tamped down and subsequently tracked over to push them into the underlying peat. Despite the apparent physicality of disturbance to the soil surface, vibrations from groundworks were not felt beyond the reach of the excavator. The resulting peat surface in adjacent, already worked, peatland was largely bare peat, with only minor exposures of woody debris (see Figure 3.17). Fissures and cracking were conspicuous by their absence, and although the ground surface was soft, there was no evidence that significant remoulding and loss of strength had taken place within the worked peat. The primary relief was from excavator treads. Sites that had been smoothed within the previous year already showed evidence of significant vegetation regrowth, including cotton grass, and in wetter areas, some sphagnum.
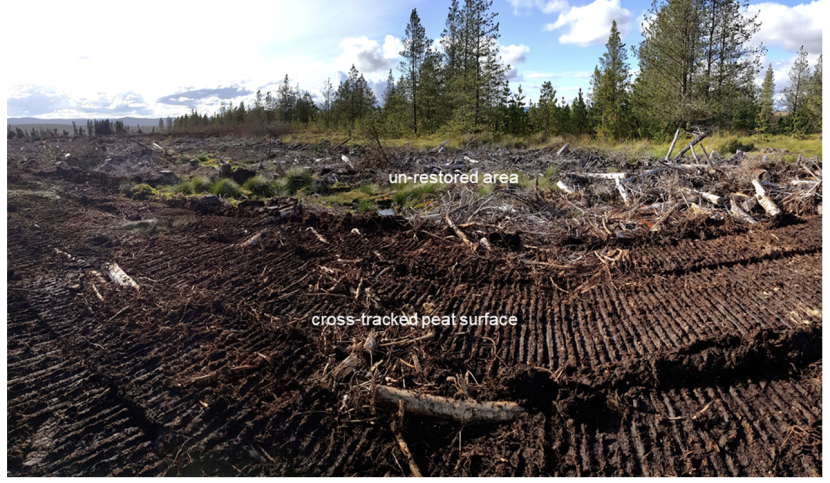
Discussion with the site contractor and FLS forester indicated that the degree of incorporation of stumps, stems and brash could vary from site to site, with all elements incorporated within the peat mass if present on the ground (for example, if an area is windthrown with loss of all stock to waste i.e. no removal of woody material). It is possible that the interlocking of brash and woody debris may provide some component of tensile strength subsequent to ground smoothing, noting that in the initial post-smoothing period (first few years) there may be a lack of other continuous vegetation cover.
3.2.6. Summary of case study findings
Key conclusions from the case study work are provided below.
For raised bogs:
- Basal slope, even if very minor, may concentrate drainage and encourage instability at the margin of the bog.
- Convergent surface and near surface (acrotelm) drainage may encourage preferential development of mosses persistently over time, reducing the tensile strength of the acrotelm in these areas.
- Peripheral trench bunding in proximity to a raised bog margin may create lines of weakness and predispose a bog margin to instability.
- Surface bunds with low elevations, dams that are not properly keyed in or constructed or trampling (by people or stock) may encourage erosion of the bog surface where these moisture retaining structures are only partially effective.
For blanket bogs:
- Drain reprofiling is effective in providing continuous vegetation cover compared with non-restored drains that would previously have lacked a continuous tensile surface – this effect is likely to improve with time.
- Interception of peat pipes by reprofiling can block pipes and cause waterlogging / ponding where they intersect; more monitoring of these effects is required to provide evidence of implications for ground stability but it is likely that pipe blocking may reduce stability.
- Un-restored drains have been demonstrated to influence the occurrence of instability, however, no such effect has been demonstrated for restored drains.
- Evidence of ground disturbance in the form of track marks and loss of vegetation from plant trafficking is minimal in the short to medium term.
- Reprofiling works close to bog margins or steep slopes (e.g. large gully or valley sides) should be minimised to avoid trafficking of plant on marginally stable peat.
For afforested bogs:
- Ground-smoothing is highly effective in reverting an undulating afforested bog surface (ridge and furrows / drains) to a surface with low relief, however, it removes any surface tensile strength component remaining within the understorey vegetation (which is disrupted by the ground smoothing process).
- This loss of tensile strength may be compensated for by the incorporation of woody debris into the peat mass, including root plates, stems and brash, though typically on a successfully afforested site, only stumps would be incorporated (as brash and stems would be harvested and/or removed from site).
- The use of low ground pressure excavators minimises point loads on the peat bog and there have been no incidences of excavator loss or ground collapse during ground smoothing work.
- While operators reported that most slope ranges could successfully be subject to ground smoothing, monitoring is recommended to ensure that such works do not reduce the stability of slopes (case study sites were very gently or neutrally sloping).
It should be noted that these findings are drawn from a relatively limited number of case study examples. In the event that further episodes of instability occur in association with restoration, it is strongly recommended that post-instability field inspection and analysis is undertaken to identify any further relationships between restoration and ground movements.
3.3. Experience of Peatland ACTION officers
In addition to the desk-based review of Peatland ACTION sites and field-based case studies, the experience of Peatland ACTION officers and wider practitioners was sought as follows:
- Through provision of a training session to the Peatland ACTION team at the project inception meeting, providing a detailed overview of peat instability as a phenomenon (while also providing an opportunity for feedback).
- Through site-based discussion of restoration techniques with Peatland ACTION officers in both blanket bog and raised bog settings and in afforested and non-forested environments.
- Through discussion with an experienced plant operator working on the FLS trial plots and discussion with a highly experienced forest restoration practitioner.
- Through presentation of a poster drawing attention to the project objectives at the 2019 IUCN UK Peatland Programme meeting in Belfast with the intent of raising the profile of the project and soliciting feedback from practitioners across the UK and Ireland (see Annex 1).
During discussions with Peatland ACTION officers, the following points were made in relation to the protocols and wider issues around restoration:
- The protocols should be straightforward to use, with minimal requirement for technical knowledge of peat instability but with a suitable technical audit trail to explain their structure and implementation (should it be required).
- Information on peat geomorphology other than peat instability would be helpful to understand whether features are instability related or not (particularly erosion features such as gullies).
- It was appreciated that monitoring of sites post-restoration would be helpful to compile an evidence base for i) the effectiveness of restoration, and ii) identifying any unanticipated negative impacts of restoration, however funding timescales (already challenging for enabling restoration work due to a 12 month funding cycle) did not currently allow this.
- Information on how to monitor sites that have already failed (e.g. Mossband and Greenhead Moss) would be helpful to provide early warning of any future recurrence or deterioration.
The next chapter provides a detailed overview of how baseline landslide susceptibility can be calculated for blanket bogs and raised bogs, and how the effects of restoration on this baseline susceptibility can be assessed.
4. Approach to identifying potential effects of restoration on peat stability
4.1. Introduction
Prior to restoration, a peatland site will have a baseline level of natural stability. This will reflect the ground conditions at the site, including physical and hydrological characteristics of the peat, slope morphology, geomorphological condition and degree of human modification. Restoration groundworks have the potential to modify this baseline stability by changing some of these ground conditions.
In landslide risk assessment, there are a range of approaches to assessing natural stability of soils and the effects of human activities. Qualitative approaches typically use weighted factors of relevance to ground conditions, where factors are selected according to known controls on stability (e.g. peat depth, slope angle, substrate geology, etc). Quantitative approaches use representative numerical parameters (e.g. soil strength, water table depth, slope angle) to test the stability of a soil, and provide a numerical estimate of stability.
Approaches to slope stability analysis in relation to peat are described in the Scottish Government (2017) Best Practice Guidance (BPG), and although that guidance focuses on energy developments, the assessment of baseline stability is no different for peatlands due for restoration. The effects of restoration groundworks on stability are quite different however, and this is considered further below.
4.2. Landslide susceptibility approach
The qualitative approach used to support the risk-based protocols in this study aligns with the Scottish Government (2017) BPG, using a ranking system that relates ground conditions to the probability of landslide occurrence, e.g. the presence or absence of instability features at the site, or combinations of scored ‘hazard factors’ (e.g. slope, peat depth, orientation of slope drainage) whereby higher scores indicate higher probability of future peat landslides.
In order for the approach to be credible, where expert judgement is used instead of measured information, judgements should be transparent through full documentation of sources of evidence, and the logic behind any factoring or scoring approach should be clearly detailed. The assessment of baseline (or pre-restoration) peat landslide susceptibility considers the following ground conditions:
- Peat depth (P).
- Slope angle (S).
- Slope form (convexity, concavity) (C).
- Substrate geology (G).
- Peat morphology (M).
- Peatland drainage features (D).
- Forestry (F).
- Land use (L).
The susceptibility approach evaluates ground conditions across a site that may contribute to peat instability. When undertaking detailed site analysis to support local decision making (e.g. within a small subarea of a site), the variability in these factors would typically be mapped in some detail within the site boundary. Locations within a site with the highest concentration of adverse ground conditions will be most susceptible to instability, and those with few adverse ground conditions would be the most stable. This form of analysis would typically be undertaken by geoscience specialists (geomorphologists, geologists or geotechnical specialists) with detailed knowledge of slope stability. For this study, the approach must reflect the expertise of the Peatland ACTION officers and the status of peat stability as just one of a series of criteria being used to evaluate the suitability of sites for restoration groundwork. Accordingly, ground conditions are more appropriately considered as site averages or representative estimates.
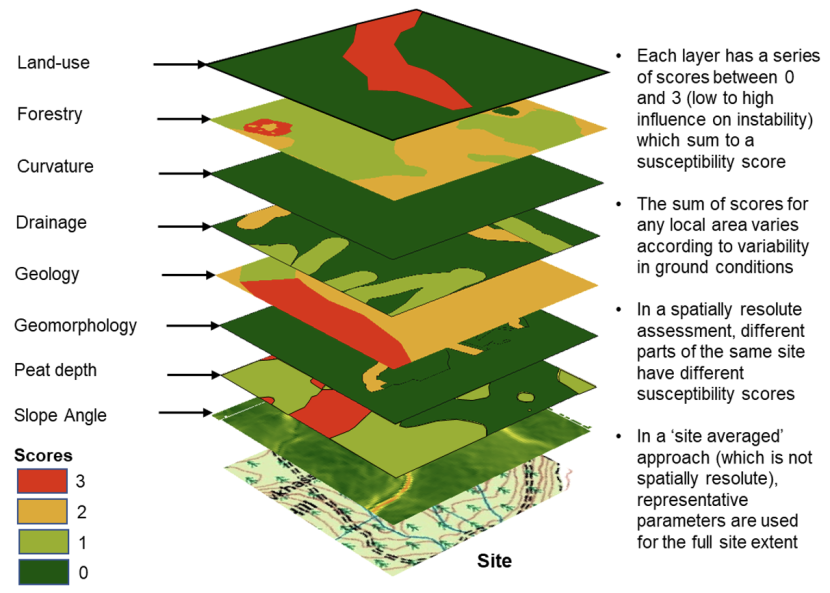
A distinction is made between spatially resolute and site averages – in the former a site may be subdivided into many small compartments based on differing combinations of the eight factors at any individual location, in a site averaged approach, a single value is assumed for each factor across the site.
Each ground condition is reviewed in terms of its influence on instability, and assigned a score between 0 (no influence) and 3 (greatest influence). The higher a score, the greater the contribution of that factor to instability for any particular site. Scores are summed for each site to produce a peat landslide susceptibility score SPL, where the suffixes of the contributory factors relate to the ground conditions listed above:
SPL = SP + SS + SC + SG + SM + SD + SF + SL
The theoretical maximum score for any site is therefore 24, although the chance of all adverse ground conditions being present in any one site is small.
The following sections consider the influence of each factor on instability. The peat landslide database and review of Peatland ACTION sites is used as an evidence base for the scores assigned to each factor. Because blanket bogs and raised bogs differ in morphological setting, the approach to landslide susceptibility differs in each case. Peat slides and bog bursts are scored separately, since they have different associations with peat depth and slope angle (in particular) and are therefore often occur in different settings.
4.2.1. Influence of ground conditions on stability in blanket bogs
4.2.1.1. Peat depth (P)
Table 4.1 shows peat depth ranges, their influence on stability and related scores for the peat depth ground condition. Peat depths should be derived from site specific probing data and scoring reflects the peat depth ranges most frequently associated with peat slides and bog bursts (from the updated database, see Figure 2.7).
Table 4.1. Peat depth classes, influence on stability and scores
Depth range (m) |
Significance |
Score (Peat Slide) |
Score (Bog Burst) |
---|---|---|---|
>1.5 |
Bog bursts are the dominant failure mechanism in this depth range, peat slides rarely occur |
1 |
3 |
1.0 - 1.5 |
Peat slides are the dominant failure mechanism in this depth range, bog bursts rarely occur |
3 |
1 |
0.5-1.0 |
Peat slides are the dominant failure mechanism in this depth range, bog bursts do not occur |
2 |
0 |
<0.5 |
Organic soil rather than peat, failures would be peaty-debris slides |
0 |
0 |
Peat typically becomes deeper as slope angles decrease, and the peat may become more distinctly layered and more humified at its base. As a result, the failure mechanism may change from peat slides in shallower peats to bog bursts in deeper peat.
4.2.1.2. Slope angle (S)
Table 4.2 shows slope angle ranges, their influence on stability and related scores for the slope angle ground condition. Slope angles can be derived from elevation plots within Google Earth ProTM, from site specific digital terrain data or from contour plots on Ordnance Survey maps. Typically, landslides in peat covered slopes transition from peaty-debris slides (which are not true peat landslides) on steep slopes, to peat slides on moderate slopes and bog bursts on shallow slopes (see Figure 2.7).
Table 4.2. Slope angle classes, influence on stability and scores
Slope range (°) |
Significance |
Score (Peat Slide) |
Score (Bog Burst) |
---|---|---|---|
>15 |
Failure typically occurs as peaty-debris slides or peat slides, although to a lesser extent than on gentler slopes, bog bursts rarely occur |
3 |
1 |
7.5 - 10.0 |
A frequently reported slope range for peat slides, bog bursts sometimes occur in this slope range |
3 |
1 |
5.0 – 7.5 |
A frequently reported slope range for peat slides, bog bursts are also reasonably common in this slope range |
3 |
2 |
0 - 5.0 |
The most frequently reported slope range for bog bursts, though peat slides may still occur |
1 |
3 |
4.2.1.3. Slope form (C)
Table 4.3 shows slope form classes, influence on stability and related scores. Slope form can be interpreted from elevation plots in Google Earth ProTM or from changes in contour spacing on Ordnance Survey maps. Convex and concave slopes (i.e. positions in a slope profile where slope gradient changes by a few degrees) have been associated with the initiation point of peat landslides by a number of authors. Convexities are often associated with thinning of peat, such that thicker peat upslope applies stresses to thinner ‘retaining’ peat downslope. Conversely, buckling and tearing of peat may trigger failure at concavities (e.g. Dykes and Warburton, 2007a; Boylan et al., 2011).
However, landslide database work undertaken for this study indicates that peat slides generally occur on rectilinear (straight in profile) slopes, or convex slopes, while bog bursts tend to occur on convex slopes and to a lesser extent on rectilinear slopes (see Figure 2.7). No peat failures were identified within areas of undulating peat among rock outcrops.
Table 4.3. Slope form classes, influence on stability and scores
Profile Convexity |
Significance |
Score (Peat Slide) |
Score (Bog Burst) |
---|---|---|---|
Rectilinear Slope |
Peat slides are most frequently reported on rectilinear slopes; bog bursts are often reported on rectilinear slopes |
3 |
2 |
Convex Slope |
Both peat slides and bog bursts are often reported on or above convex slopes |
2 |
3 |
Concave Slope |
Peat failures are occasionally reported in association with concave slopes |
1 |
1 |
Undulating |
Peat failures are rarely reported in areas of undulating peat set amongst rock outcrops |
1 |
1 |
4.2.1.4. Substrate geology (G)
Table 4.4 shows substrate geology, influence on stability and related scores for peat depth. Substrate geology can be derived from British Geological Survey online viewers, from feedback on substrate from peat depth probe surveys (which are required ahead of Peatland ACTION funding applications) or from observations of substrate made during site survey.
The shear surface or failure zone of peat failures typically overlies an impervious clay or mineral (bedrock) base giving rise to impeded drainage (Warburton et al., 2004). This, in part, is responsible for the presence of peat, but also precludes free drainage of water from the base of the peat mass, particularly under extreme conditions (such as after heavy rainfall, or snowmelt).
Table 4.4. Substrate geology classes, influence on stability and scores
Substrate Geology |
Significance |
Score (Peat Slide) |
Score (Bog Burst) |
---|---|---|---|
Cohesive (clay) or iron pan |
Failures are often associated with clay substrates and/or iron pans |
3 |
3 |
Gritty / granular till with clay |
Failures are occasionally associated till substrates with a minor clay component |
2 |
2 |
Unknown |
Lack of understanding of substrate condition is a significant uncertainty (an intermediate score is therefore applied) |
2 |
2 |
Granular or bedrock |
Failures are less frequently associated with bedrock or granular (silt / sand / gravel) substrates |
1 |
1 |
Peat failures are frequently cited in association with glacial till deposits in which an iron pan is observed in the upper few centimetres (Dykes and Warburton, 2007a). They have also been observed over glacial till without an obvious iron pan, or over impermeable bedrock. They are rarely cited over permeable bedrock, probably due to the reduced likelihood of peat formation
4.2.1.5. Peat morphology (M)
The geomorphological condition of a peatland may indicate past or potential instability. Morphology can be determined from review of satellite imagery on Google Earth ProTM.
Sites with minimal erosion and extensive areas of planar peat may have well developed pipe networks or continuous well stratified peat profiles that may enable development of shear surfaces or failure zones over larger areas. In contrast, peat deposits draping undulating rocky topography or sites that are heavily eroded / gullied may be compartmentalised and less susceptible to large-scale failure. In addition, instability indicators such as collapsed pipes, or tension cracks may indicate a predisposition towards instability.
Table 4.5 shows peat morphology classes, their influence on stability and related scores for the peat morphology factor. Note that this table allows pre-existing peat landslides to generate a score of 4. This reflects the observation on Figure 2.9 that peat landslides often occur on slopes that have previously been affected by instability.
Table 4.5. Peat morphology classes, influence on stability and scores
Geomorphology |
Significance |
Score (Peat Slide) |
Score (Bog Burst) |
---|---|---|---|
Pre-existing peat landslide |
Failures often recur on hillslopes with pre-existing peat landslides |
4 |
4 |
Planar with pipes |
Failures generally occur on planar slopes, and are often reported in areas of piping |
3 |
3 |
Planar with pools |
Bog bursts are more likely in areas of perched water or subsurface water bodies (pools that have vegetated over) |
2 |
3 |
Planar (no other features) |
Failures generally occur on planar slopes where subsurface hydrology is intact |
2 |
2 |
Undulating peat within areas of numerous rock outcrops |
Failures are rarely reported in areas with significant rock outcrops where there is a lack of laterally continuous geotechnical conditions |
1 |
1 |
Slightly eroded |
Failures are rarely reported in areas with occasional gullying and/or bare peat (<20% of an area) |
1 |
1 |
Heavily eroded |
Failures are not reported in areas that are heavily eroded or bare (>20% of an area) |
0 |
0 |
No geomorphology (afforested) |
Peatland disrupted by forestry lacks the subsurface hydrology to cause instability (forestry effects are considered separately) |
0 |
0 |
4.2.1.6. Peat drainage (D)
Table 4.6 shows artificial and natural drainage feature classes, their significance and related scores. The presence of drains and their orientation can be derived from inspection of satellite imagery and Ordnance Survey data. Transverse / oblique drainage lines, both natural and artificial, may reduce peat stability by creating lines of weakness in the peat slope and encouraging the formation of peat pipes. A number of peat failures have been identified which have failed over moorland grips (Warburton et al., 2004).
Table 4.6. Peat drainage classes, influence on stability and scores
Drainage Feature |
Significance |
Score (Peat Slide) |
Score (Bog Burst) |
---|---|---|---|
Drains are generally aligned along contours (<15°) |
Only peat slides have been observed in association with contour aligned drains; hypothetically, contour aligned drains have greatest effect on instability |
3 |
3 |
Drains are generally aligned oblique (15°- 60°) to contour |
Most reports of peat slides and bog bursts in association with drainage occurs where drains are oblique to slope |
2 |
2 |
Drains are generally aligned downslope (<30° to slope) |
Failures are rarely associated with artificial drains parallel to slope or adjacent to natural drainage lines |
1 |
1 |
No / minimal artificial drainage |
No influence on stability |
0 |
0 |
In theory, the influence of changes in hydrology becomes more pronounced the more transverse the orientation of the drainage lines relative to the overall slope. This is partially supported by peat landslide database work undertaken for this study (see Figure 2.7). Roughly 40% of peat slides and 30% of bog bursts occur in drained areas, though usually in association with drains that are oblique to slope rather than along contour (noting that contour aligned drains are less common).
4.2.1.7. Forestry (F)
Table 4.7 shows forestry classes, their influence on stability and related scores. Forestry condition can be derived from satellite imagery and Ordnance Survey data.
Table 4.7. Forestry conditions, influence on stability and scores
Forestry Class |
Significance |
Score (Peat Slide) |
Score (Bog Burst) |
---|---|---|---|
Afforested area with rows generally oblique to slope |
Peat underlying forestry stands with rows aligned oblique to slope has inter ridge cracks which are conducive to slope instability |
2 |
2 |
Afforested area with rows generally aligned to slope |
Peat underlying forestry stands with rows aligned with slope is conducive to slope instability, but less so than where rows are aligned oblique to slope |
1 |
1 |
Deforested area with rows generally oblique to slope |
Peat underlying deforested stands has a higher water table and more neutral buoyancy, but retains inter ridge cracks (lines of weakness) conducive to instability; alignment of cracks oblique to slope is most conducive to instability |
3 |
3 |
Deforested area with rows generally aligned to slope |
Peat underlying deforested stands has a higher water table and more neutral buoyancy, but retains inter ridge cracks (lines of weakness), however, orientation of these cracks is less critical when aligned to slope |
2 |
2 |
Not afforested |
No influence on stability |
0 |
0 |
A report by Lindsay and Bragg (2005) on Derrybrien suggested that row alignments, desiccation cracking and loading (by trees) could all influence peat stability. Review of peat landslides as part of the database work for this report indicates that a very small proportion of the recorded landslide population has occurred within forestry (see Figure 2.7). However, forestry activities have effects in common with drainage modifications, and these, alongside hypothesised effects are incorporated in Table 4.7.
4.2.1.8. Land use (L)
Table 4.8 shows land use classes, their influence on stability and related scores. A variety of land uses have been associated with peat failures (see Section 2.2.4). Review of peat landslide locations as part of this work indicates that none of the land uses implicated in causation of peat landslides are commonly associated with them. Given this, the influences on stability shown in Table 4.8 are derived from credible physical principles rather than evidence, e.g. the effects of cuttings on adjacent, upslope peat (which might act to reduce its stability) or the effects of machine cutting in fragmenting the peat mass (not dissimilar to the effects of cutting of drains).
Table 4.8. Land use, influence on stability and scores
Land Use |
Significance |
Score (Peat Slide) |
Score (Bog Burst) |
---|---|---|---|
Machine cutting |
Machine cutting of peat has the most significant impacts on slope compartmentalisation, but has been reported primarily in association with peat slides |
3 |
2 |
Hand cutting (turbary) |
Hand cutting may remove slope support from adjacent upslope materials (albeit slowly) and has been reported in associated with failure of raised bogs |
1 |
2 |
Burning (deep, into catotelm) |
Failures are rarely associated with this activity though deep burning may create pathways for water to the base of peat (e.g. by cracking) |
2 |
2 |
Burning (shallow, within acrotelm only) |
Failures are rarely associated with burning, and with shallow burning are unlikely to create pathways to the base of the peat |
1 |
1 |
Other land use |
No influence on stability |
0 |
0 |
4.2.1.9. Calculating baseline landslide susceptibility in blanket bogs
Baseline landslide susceptibility for blanket bog sites should be calculated by summing the site average or representative scores for each scored ground condition, and then comparing the summed score with the score ranges and susceptibility ratings in Table 4.9.
Table 4.9. Summed susceptibility score ranges for blanket bogs
Summed Score from Contributory Factors |
Typical site conditions associated with score |
Likelihood (qualitative) |
---|---|---|
< 8 |
Unmodified peat with no more than low weightings for peat depth, slope angle, underlying geology and peat morphology |
Very Low |
8 - 13 |
Unmodified or modified peat with no more than moderate or some high scores for peat depth, slope angle, underlying geology and peat morphology |
Low |
14 - 18 |
Unmodified or modified peat with high scores for peat depth and slope angle and / or high scores for at least three other contributory factors |
Moderate |
19 - 21 |
Modified peat with high scores for peat depth and slope angle and several other contributory factors |
High |
> 21 |
Modified peat with high scores for most contributory factors (unusual except in areas with evidence of incipient instability) |
Very High |
For a site to have a moderate or higher likelihood of a peat landslide, a likelihood score would be required equivalent to both the worst case peat depth and slope angle scores (3 in each case, i.e. 3 × 2 classes) alongside three intermediate scores (of 2, i.e. 2 × 3 classes) for other ground conditions. This means that any likelihood score of 14 or greater would be equivalent to at least a Moderate baseline likelihood of a peat landslide under appropriate trigger conditions, such as unusually intense rainfall. Given that the maximum score attainable is 24, this seems reasonable.
4.2.2. Influence of ground conditions on stability in raised bog
In contrast to blanket bogs, there are relatively few recent landslides reported in raised bogs, and an evidence base from which to derive guiding principles for susceptibility analysis is limited. The majority of the previously reported landslides occurred pre-1900, and these failures could not be located in the review of satellite imagery due to the degree of disturbance to these peatlands since that time.
Of the eight ground conditions considered applicable to blanket bog, substrate geology, peat morphology and forestry are carried forward into the assessment of raised bogs. The remaining five classes are modified as detailed in the sections below. Only bog bursts are considered for raised bogs as no peat slide morphology has been reported in these areas of minimal slope.
4.2.2.1. Peat depth (PRB)
The deeper the raised bog, the greater the likelihood of failure since the degree of humification of the basal layers will likely be higher and the proportion of its depth held together by the tensile acrotelm and underlying fibrous layers will be less. There is limited information on the depth of peat associated with raised bog failures.
As with blanket bogs, site-specific peat depth data should be acquired where possible. If this is not achievable, the James Hutton Institute hosts a database of peat survey data for peat deposits in Scotland (JHI, 2020), a link to which is provided in the reference list of this report.
The two case studies from this work (Greenhead Moss and Mossband) occurred in bogs with dome thicknesses of 3.5m and 4.5m. Of the two historical reported “moss” failures, Solway Moss (1771) was reported as c. 7.6m in thickness and Chat Moss (1600s) as c. 8m in thickness. Two more recent failures in Irish raised bogs (Long et al., 2014) occurred in raised bogs up to 8m in thickness.
Accordingly, a linear scale is applied for peat depth up to a credible upper thickness of 10m, as shown in Table 4.10.
Table 4.10. Peat depth classes for raised bog, influence on stability and scores
Depth range (m) |
Significance |
Score (Bog Burst) |
---|---|---|
>6 |
Very deep raised bogs will be the most humified and may have significant relief at their margins or significant doming. All published raised bog failures of significant scale occurred in this range. |
3 |
4 – 6 |
Deep raised bogs will be very humified and may have significant doming. One of two case study raised bog failures occurred in this depth range. |
2 |
2 – 4 |
Moderate depth raised bogs will be more humified and may have some doming. One of two case study raised bog failures occurred in this depth range. |
1 |
0 – 2 |
Shallow raised bogs will be less humified and have minimal relief. No failures have been reported in raised bogs of this depth range. |
0 |
4.2.2.2. Slope angle (SRB)
In contrast to blanket bogs, raised bogs typically accumulate on flat terrain and usually have relatively neutral slopes on their bog surfaces. Both the Mossband and Greenhead Moss raised bogs visited for this study and the two raised bog failures investigated by Long et al. (2014) have basal slopes which dip very gently towards failure locations. Accordingly, a simple classification of base slope is indicated in Table 4.11. Base slope can be calculated by comparing peat depth measurements with digital elevation data for the bog surface on transects across the bog.
Table 4.11. Basal slope classes for raised bogs, influence on stability and scores
Raised bog basal slope |
Significance |
Score (Bog Burst) |
---|---|---|
Inclined |
All recorded raised bog failures have minor dipping basal slopes towards the areas that have experienced failure |
3 |
Neutral |
Neutral basal slopes (flat) are not expected to influence failure |
0 |
4.2.2.3. Slope form (CRB)
Again, in contrast to blanket bogs, raised bogs are relatively flat and the primary characteristic of slope form is the degree of doming. It is hypothesised that higher domes may have a greater degree of lateral stress than very gentle domes due to the volume to surface ratio of the bog – a very ‘domed’ bog will have more peat ‘held in’ by less tensile surface material, while also being steeper at the margins. Given this, a simplistic classification is provided in Table 4.12.
Table 4.12. Slope form (or dome shape) classes for raised bogs, influence on stability and scores
Slope form (dome shape) |
Significance |
Score (Bog Burst) |
---|---|---|
≥2° |
It is likely (though unreported) that higher domes may be less stable than shallower domes |
3 |
1° to 2° |
Most reported raised bog failures occur where the mean gradient from the dome high to the toe of the blanket margin is between 1 and 2° |
2 |
<1° |
Non-domed, i.e. flat raised bogs are the least likely to experience failure due to their negligible gradients |
1 |
4.2.2.4. Peat drainage (DRB)
Case study evidence from both raised bogs visited as part of this study indicates that surface drainage may have played a significant role in determining both the occurrence and location of failure (see Section 3.2.6).
Convergent drainage towards a low point on the bog surface may increase moisture content in that area where drains fail to transmit water beyond the bog margin. They may also encourage a longer term tendency towards wetter, less tensile surface vegetation (e.g. abundance of mosses), evident both at the contemporary ground surface and in the accumulated stratigraphy of the peat. The presence of peripheral drains (running around the circumference of the bog) may also act to weaken the tensile layer at the margins, where slopes may be greater and where instability tends to preferentially occur through margin failure. Accordingly, Table 4.13 shows classes, significance and scores for peat drainage for raised bogs
Table 4.13. Peat drainage classes for raised bogs, influence on stability and scores
Peat drainage class |
Significance |
Score (Bog Burst) |
---|---|---|
Peripheral + organised |
Presence of peripheral drain and presence of convergent drainage towards lowest area of raised bog (worst case) |
3 |
Peripheral + disorganised |
Presence of peripheral drain and disorganised drainage (intermediate case) |
2 |
Peripheral |
Presence of peripheral drain and no other drainage (intermediate case) |
2 |
Organised |
Presence of convergent drainage towards lowest area of raised bog (intermediate case) |
2 |
Disorganised |
Presence of disorganised drainage (least effect case) |
1 |
None |
No drainage (no effect) |
0 |
4.2.2.5. Land use (LRB)
Land use in raised bogs is similar to that in blanket bogs, primarily comprising forestry (often alongside drainage) and cutting. Cutting often occurs around the periphery of raised bogs and over long periods, steepening their margins, removing the toe of the slope and encouraging preferential drainage towards any cut faces. One of the case study examples for this study (Greenhead Moss) and one raised bog failure reported by Long et al. (2014) occurred in areas that had been cut.
Table 4.14 provides classes, significance and scores for land use on raised bogs. Land use features are typically visible on satellite imagery, historical map data or may be determined from historical information from national or local conservation groups.
Table 4.14. Land use classes for raised bogs, influence on stability and scores
Land Use |
Significance |
Score (Bog Burst) |
---|---|---|
Cutting |
May remove support from adjacent peat and encourage preferential subsurface flows towards the cut face. |
3 |
Cutting and plantation forestry |
May remove support from adjacent peat which has been modified by effects of plantation forestry and drainage. |
3 |
Plantation |
Reduces surface tensile strength and may encourage preferential drainage towards low points in the raised bog surface depending on row alignment |
2 |
Informal forestry |
Locally reduces surface tensile strength, no systematic effect on drainage. |
1 |
Burning (deep, into catotelm) |
Failures are rarely associated with this activity though deep burning may create pathways for water to the base of peat (e.g. by cracking) |
2 |
Burning (shallow, within acrotelm only) |
Failures are rarely associated with burning, and with shallow burning are unlikely to create pathways to the base of the peat |
1 |
No land Use |
No influence on stability. |
0 |
4.2.2.6. Calculating baseline landslide susceptibility in raised bogs
The score ranges for raised bogs are shown in Table 4.15.
Table 4.15. Summed susceptibility score ranges for raised bogs
Summed Score from Contributory Factors |
Typical site conditions associated with score |
Likelihood (qualitative) |
---|---|---|
< 8 |
Normal conditions for a shallow raised bog, unmodified with potential for doming, no basal slope and no other distinct morphology |
Very Low |
8 - 11 |
Normal conditions for a moderately deep raised bog, some doming, some drainage, no basal slope, some pooling |
Low |
12 - 14 |
Modified conditions in moderate to deep bog with one or more ground conditions with worst case scores (e.g. with an inclined base and peripheral drainage) |
Moderate |
15 - 19 |
Modified conditions in moderate to deep raised bog with two or more ground conditions with worst case scores |
High |
> 19 |
Heavily modified conditions in deep bog with three or more ground conditions with worst case scores |
Very High |
4.3. Geotechnical and geomechanical approaches to quantifying effects
4.3.1. Infinite Slope Analysis
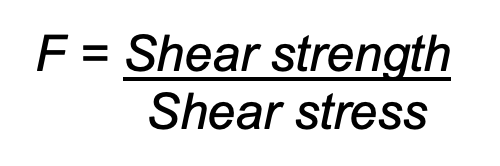
The likelihood of a particular slope or hillside failing can also be expressed as a Factor of Safety (F). For any potential failure surface, there is a balance between the weight of the potential landslide (driving force or shear stress) and the strength of the peat and/or its contact with the substrate on the hillside (shear resistance). Provided the available shear resistance is greater than the shear stress then the Factor of Safety will be greater than 1.0 and the slope will remain stable. If the Factor of Safety reduces to less than 1.0 through a change in ground conditions e.g. through the application of a trigger, the slope will fail.
The shear stress is mostly a component of the weight of the peat making up the potential landslide mass. The shear resistance is provided by the frictional and tensile strength of the peat and/or substrate, and the normal effective stress and influence of groundwater changing the normal load (weight) of the sliding mass (Warburton et al., 2004; Dykes and Warburton, 2007b; Dykes and Warburton, 2008).
Where stability analysis has been applied to peat slopes (e.g. Carling, 1986; Warburton et al., 2004; Dykes and Warburton, 2008), the infinite slope model has provided the most informative results. The infinite slope model assumes a planar translational failure, where the shear surface is parallel to the ground surface, and the length of the slope is large in comparison to the failure depth (hence ‘shallow’ failure). The nature of detachment of peat landslides is most frequently by a translational mechanism, and since this is the failure type modelled by infinite slope analysis, it is the most appropriate analytical method.
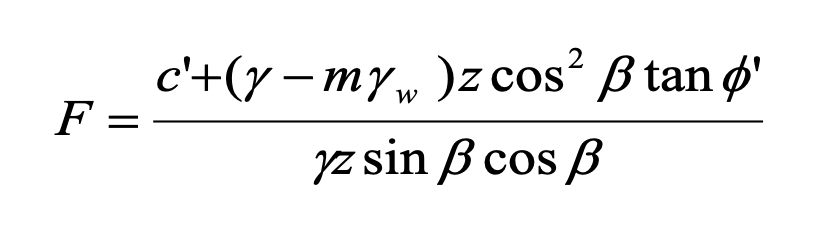
The stability of a slope can be assessed by calculating the factor of safety F, which is the ratio of the sum of resisting forces (shear strength) and the sum of the destabilising forces (shear stress): where c′ is the effective cohesion, γ is the bulk unit weight of saturated peat, γw is the unit weight of water, m is the height of the water table as a fraction of the peat depth, z is the peat depth in the direction of normal stress, β is the angle of the slope to the horizontal and ϕ′ is the effective angle of internal friction.
Values of F < 1 indicate a slope would have undergone failure under the conditions modelled; values of F > 1 suggest conditions of stability.
4.3.2. Uncertainties
There are significant uncertainties in the reliability of geotechnical testing used to characterise peat shear strength, and also issues around the validity of applying conventional slope stability analysis to peat soils. The latter arises from the physical composition of peat, which is a composite of plant structures in various states of decay and water (some held within plant structures and some in the pores). Soil mechanics is predicated on frictional contacts between soil particles with averaged pore size and spacing. None of these criteria are met by peat.
Nevertheless, in the absence of alternative approaches specific to organic soils, most academics and professionals continue to apply the infinite slope model to the assessment of peat stability, and use geotechnical parameters derived from conventional testing. The assumption is that the governing properties of limiting equilibrium between the destabilising force of gravity on the peat mass on a sloping surface and the resisting force of the friction between the base of the peat and the substrate remain. It is also assumed that the bulk behaviour of peat as a soil will be relatively consistent for specific ranges of parameters (e.g. cohesion and angle of internal friction).
4.3.3. Application to peat restoration
For this study, geotechnical approaches have been used as follows:
- Factors of Safety have been calculated for various combinations of peat shear strength (cohesion and angle of internal friction), based on best estimate and low estimate (conservative) values derived from published literature for different types of peat (fibrous, pseudo-fibrous, amorphous), thicknesses and slope angles. The results provide a basis for identifying a hypothetical Factor of Safety (F) for any site.
- The effects of restoration groundworks have been evaluated by considering their impact on the water table, peat mass and toe support (or buttressing). This has helped justify a series of ‘restoration effect scores’ which modify baseline (pre-restoration) peat landslide susceptibility (see Section 4.4) below.
Further detail is provided in the following subsections.
4.3.3.1. Identification of geotechnical parameters for peat
Generally, input parameters for slope stability analysis are derived from site specific geotechnical data collected from site investigation and laboratory testing of representative samples. This is not practical for Peatland ACTION projects, as those applying for funding usually lack geotechnical backgrounds and would not be able to fund site investigations of the calibre required to collect the data. An alternative approach is to use parameters derived from literature review.
For the protocols, Peatland ACTION officers will be required to identify one of three categories of peat material found at the base of the peat deposits at each site. The categories are based on the degree of humification of the peat, as indicated by the von Post classification (see Table 4.16 and Annex 2). The three categories are:
- Fibrous peat, humification H3-H5.
- Pseudo-fibrous peat, humification H6-H8.
- Amorphous peat, humification H9-H10.
Most shallow to moderate depth peats (1m-2m) will typically have humification values of H7 H8 at their base, but deep peats (>3m) or very wet peats may be more amorphous (H9 H10).
While geotechnical properties of peats are difficult to determine, of those authors who have attempted to do so, most cite either a qualitative description of the peat (e.g. pseudo-fibrous peat) in association with quoted parameters, or a humification value (e.g. H8). Table 4.17 provides published parameters for peats in a variety of settings.
Table 4.16. The modified von Post classification (after Hobbs, 1986)
Degree of humification |
Decomposition |
Plant structure |
Content of amorphous material |
Material extruded on squeezing (passing between fingers) |
Nature of residue |
---|---|---|---|---|---|
H1 |
None |
Easily identified |
None |
Clear, colourless water |
- |
H2 |
Insignificant |
Easily identified |
None |
Yellowish water |
- |
H3 |
Very slight |
Still identifiable |
Slight |
Brown, muddy water, no peat |
Not pasty |
H4 |
Slight |
Not easily identified |
Some |
Dark brown, muddy water, no peat |
Somewhat pasty |
H5 |
Moderate |
Recognisable, but vague |
Considerable |
Muddy water and some peat |
Fibres and root more resistant to decomposition |
H6 |
Moderately strong |
Indistinct (more distinct after squeezing) |
Considerable |
About one third of peat squeezed out |
Fibres and root more resistant to decomposition |
H7 |
Strong |
Faintly recognisable |
High |
About one half of peat squeezed out, any water very dark brown |
Fibres and root more resistant to decomposition |
H8 |
Very strong |
Very indistinct |
High |
About two thirds of peat squeezed out, also some pasty water |
- |
H9 |
Nearly complete |
Almost unrecognisable |
- |
Nearly all the peat squeezed out as a fairly uniform paste |
- |
H10 |
Complete |
Not discernible |
- |
All the peat passes between fingers, no free water visible |
- |
Table 4.17. Published geotechnical parameters for peats in both blanket bog and raised bog settings
Parameter |
Values used in stability analysis |
Rationale |
Source |
---|---|---|---|
Effective cohesion (c') [kPa] |
2 (amorphous) 3 (pseudo-fibrous) 5 (fibrous) |
Credible conservative cohesion values for humified peat based on literature review |
5.5 - 6.1, peat type not stated (Long, 2005) 3, 4, peat type not stated (Long, 2005) 5, basal peat (Warburton et al., 2003) 8.74, fibrous peat (Carling, 1986) 4, peat type not stated (Dykes and Kirk, 2001) 7 - 12, H8 peat (Huat et al., 2014) |
Bulk unit weight (ү) [kN/m3] |
10.5 |
Credible mid-range value for humified catotelmic peat |
10.8, catotelm peat (Mills, 2002) 10.1, Irish bog peat (Boylan et al., 2008) |
Effective angle of internal friction (ϕ') [°] |
25 (amorphous), 30 (pseudo-fibrous) 35 (fibrous) |
Credible conservative friction angles for humified peat based on literature review |
40 - 65, fibrous (Huat et al., 2014) 50 - 60, amorphous (Huat et al., 2014) 36.6 - 43.5, peat type not stated (Long, 2005) 31 - 55, Irish bog peat (Hebib, 2001) 34 - 48, fibrous sedge pear (Farrell and Hebib, 1998) 32 - 58, peat type not stated (Long, 2005) 23, basal peat (Warburton et al., 2003) 21, fibrous peat (Carling, 1986) |
The specific parameters used are c’ (effective cohesion) and ϕ’ (angle of internal friction). Cohesion is the ‘stickiness’ of the material, e.g. clays are cohesive materials and sands are not. Usually cohesion is a minor component of peat’s strength. The angle of internal friction describes the internal resistance of the soil structure to movement of its particles. Typically, angular particles (such as sand) would have a higher ϕ’ than clays since it is usually particle shape that dominates this parameter. Peats are highly variable materials and may have significant roughness when undecomposed but behave much more like a fine silt (or even clay) when very humified or amorphous.
4.3.3.2. Scenario testing
Peat geotechnical parameters were combined with other parameters to test a number of restoration scenarios geomechanically. The scenarios tested were as follows:
- Effect of water table (h) change on stability.
- Effect of toe buttressing on stability (where buttressing is the addition of support to upslope material, e.g. through construction of dams, reprofiling or infilling).
- Effect of removal of trees on stability (including water table rebound and loss of mass of the tree stock).
Table 4.18 shows the parameter ranges and justifications for each scenario.
Table 4.18. Input parameters to testing of restoration scenarios using slope stability analysis
Parameter |
Values |
Rationale |
---|---|---|
Furrow depth [m] |
0.5 |
Typical value based on site visit observations |
Crack depth [m] |
0.5 |
Typical value, based on Lindsay and Bragg (2005) observation that cracks can extend up to 70cm |
Maximum water table draw-down depth [m] |
1.0 |
Typical value based on site visit observations |
Average pressure exerted on ground by tree [kPa] |
1.1 |
Assumes a 15m tall tree with a 0.2m diameter trunk and a typical density of 0.35 g/cm3 (Sitka Spruce) and an average tree density of 0.4 trees per metre |
The results of the scenario testing in terms of the effects of each restoration technique on the stability of blanket bogs and raised bogs are considered further in the next section.
4.4. Assessment of effects of restoration techniques
This section considers the potential effects of each restoration technique on peat stability. Effects are considered in terms of weighting of factors in the susceptibility approach and modification to input parameters in the Factor of Safety (or geotechnical) approach. For each technique, the following information is presented:
- Objective of technique with regard to change in peatland condition (which may be a hydrological objective, ecological objective or other).
- Summary of groundworks associated with technique as relevant to ground stability.
- Description of physical and hydrological effects of restoration techniques.
- Definition of post-restoration modifying scores (or restoration effect scores) for the susceptibility approach (informed by geomechanical principles where possible).
The resulting post-restoration modified susceptibility is equivalent to:
Baseline susceptibility score + Restoration effect score
The aims and methods for each technique are summarised from more detailed descriptions provided in an internal Peatland ACTION technical compendium document (version 6, May 2019; Taylor, 2019). A version of this compendium has now been published online by NatureScot (NatureScot, 2022). The effects of each technique on ground stability are drawn from knowledge of peat failure mechanisms and the geotechnical and hydrological controls that have been discussed in this report. Other than the two raised bog failures (discussed in Section 3.2), no other landslides have been reported as a direct consequence of restoration activities.
4.4.1. Artificial drains
As one of the most widespread forms of peat modification, artificial drainage has been subject to a range of restoration techniques over a number of years. At the time of this study, there are five primary approaches to ditch (or drain) blocking, the objectives of which are to rewet the drying and exposed sidewalls of drains, reverse the adjacent drawdown of the water table and increase the residence time of water within the bog by ponding behind the dams. By blocking a closely spaced network of drains, the intention is to elevate the mean water table and encourage formation of peat forming species where species better adapted to drier conditions may have become dominant since ditches were cut.
4.4.1.1. Ditch blocking - Peat Dams
Standard peat dams are constructed of peat ‘borrowed’ from both the ditch itself and turves from adjacent ‘borrow’ pits. Dams are typically closely spaced (up to 3m) or spaced farther apart (up to 15m), with spacing reducing as slope severity increases.
Peatland ACTION note that peat dams are not effective on slopes >12° (Peatland ACTION, 2019), though they are noted to be less likely to succeed on ‘steep sites’ and require particular care in installation on slopes over 6°. Recommendations are that drains should only be restored where less than 1.5m wide and 1.2m deep, and preferably where there is still peat within the floor of the drain (i.e. it is not cut to substrate).
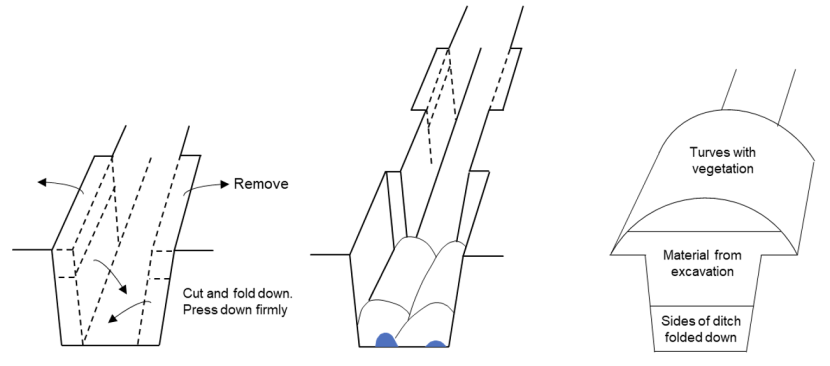
Generally, peat dams are constructed by a low ground pressure excavator, and more rarely by hand. During restoration, there will be short term removal of toe support from ditches when cutting and excavating for borrow material, while at the same time, the bog surface is subject to loading from low ground pressure (<3.5psi, 24 kPa) excavators undertaking the work.
Following restoration there may be the following physical and hydrological changes to the bog:
- The peat deposit may revert from a hydrologically and physically compartmentalised deposit to a more continuous hydrological unit, although there is potential for soil pipes crossing from one drain to another to become blocked by the peat plugs – this effect will be immediate following reprofiling
- The dams will act as plugs of peat material buttressing (providing physical support to) the upslope drain walls – this effect will require consolidation of peat in its new slope position and is unlikely to be immediate
- Water tables may rise in response to longer residence times of deeper water held between the dams (in the geomechanical approach, h will tend closer to unity, though in deeper peats the rise in water table will likely be a smaller proportion of the overall peat depth) – water table recovery is unlikely to be immediate
- There may be an associated increase in mass (due to saturation) over the same timescales as water table recovery (since it is this that drives the increase)
- The tensile strength of the acrotelm may increase across the peat deposit as a whole as transplanted turves knit together across the former drain alignment – this effect is likely to occur on a similar timescale to peat mass consolidation as it will take time for vegetation to knit across the turf boundaries.
- There will be little change in geotechnical properties of the basal peat, since it is primarily the near surface peat layers that benefit from restoration.
The peat depths recommended for this technique correspond to those in which peat slides are the primary peat failure mechanism, and are in likelihood at the lower end of the slope range within which they are observed, noting that Armstrong et al. (2009) ascribed 3° as the threshold angle above which dams should comprise artificial materials. Given this, it is likely that blanket peatlands subject to blocking with peat dams would not be particularly susceptible to peat landslides under natural conditions.
The most likely form of instability (alluded to in the NS technical compendium) is failure of the peat dams themselves along the drain axis in the event they ‘impound’ too much water for the retaining strength of the peat plug. To this end, specifications for the technique encourage cutting of depressions behind dams onto the adjacent peat surface to allow dispersal of water over the vegetated bog surface.
Restoration effect scores are summarised in Table 4.19 below:
Table 4.19. Effects of peat dams on peat stability scores
Restoration effect |
Drain orientation |
Score |
Justification |
---|---|---|---|
Slope buttressing |
Downslope |
0 |
No buttressing due to drain alignment |
Slope buttressing |
Oblique / contour |
0 |
No buttressing due to large spacing |
Tensile strength |
Downslope |
-1 |
Close spacing allows binding |
Tensile strength |
Oblique / contour |
0 |
Larger spacing minimises binding |
Water table / pore pressure |
Downslope |
+1 |
Water table rise expected |
Water table / pore pressure |
Oblique / contour |
+1 |
Water table rise expected |
Water transmission in peat pipes |
Downslope |
0 |
No pipe blocking due to drain alignment |
Water transmission in peat pipes |
Oblique / contour |
0 |
No pipe blocking assumed due to large dam spacing |
Summary: for downslope aligned drains, there will be no net destabilising effect from installation of peat dams, while for drains oblique to slope or contour-aligned there will be a net destabilising effect (a score of +1).
4.4.1.2. Ditch Blocking - Plastic Piling Dams
The objectives of plastic dam installation are the same as for peat dams. The installation technique involves driving plastic piles into the peat at the base of drains and into the banks of the ditch. No further enlarging of the ditches is required from which to borrow material. Plastic dams have greater spacing than peat dams but may require additional reinforcement as a result. The downstream side of the dams may be protected by placed materials (e.g. heather, brash) to prevent spillover undercutting the dams (see Figure 4.3). Plastic dams are recommended as terminal drains (the lowest drain in a ditch) or at the outflows for raised bogs but may not install well where the site has previously been afforested. They may bow and/or fail if not reinforced.
The following physical and hydrological changes to the bog may occur following restoration:
- The peat deposit may revert from a hydrologically and physically compartmentalised deposit to a more continuous hydrological unit; soil pipes crossing from one drain to another are unlikely to be blocked.
- The physical buttressing effect to upslope drain walls will be less than with peat dams, although the resistance to failure of the buttress itself is likely to be more than with peat dams.
- Water tables may rise in response to longer residence times of deeper water held between the dams (in the geomechanical approach, h will tend closer to unity, although in deeper peats the rise in water table will likely be a smaller proportion of the overall peat depth).
- There may be an associated increase in mass (due to saturation).
- There is unlikely to be any beneficial change in tensile strength at the bog surface.
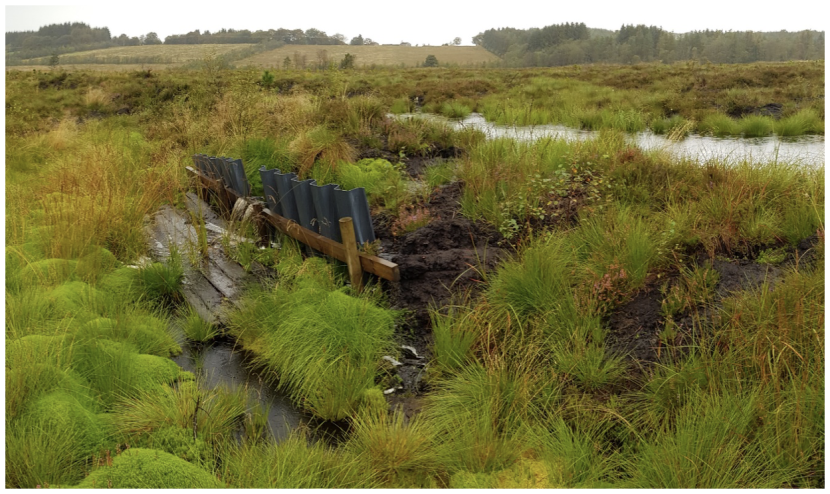
Restoration effect scores are summarised in Table 4.20 below:
Table 4.20. Effects of plastic piling on peat stability scores
Restoration effect |
Drain orientation |
Score |
Justification |
---|---|---|---|
Slope buttressing |
Downslope |
0 |
No buttressing due to drain alignment |
Slope buttressing |
Oblique / contour |
0 |
No buttressing due to large spacing |
Tensile strength |
Downslope |
0 |
Artificial structures do not encourage tensile binding |
Tensile strength |
Oblique / contour |
0 |
Artificial structures do not encourage tensile binding |
Water table / pore pressure |
Downslope |
+1 |
Water table rise expected |
Water table / pore pressure |
Oblique / contour |
+1 |
Water table rise expected |
Water transmission in peat pipes |
Downslope |
0 |
No pipe blocking due to drain alignment |
Water transmission in peat pipes |
Oblique / contour |
0 |
No pipe blocking assumed due to large dam spacing |
Summary: for all drain orientations, the overall effect of blocking using plastic piling is a minor net destabilising effect (a score of +1 in both cases).
4.4.1.3. Ditch Blocking - Wooden and Composite Dams
As ditches become larger, deeper or are set on steeper slopes, so the robustness of dam structures increases. Wooden and composite dams are often recommended for terminal drains, particularly on lowland raised bogs and for steeper slopes. An example composite dam design from the Yorkshire Peat Partnership’s Technical Guidance Notes is shown in Figure 4.4.
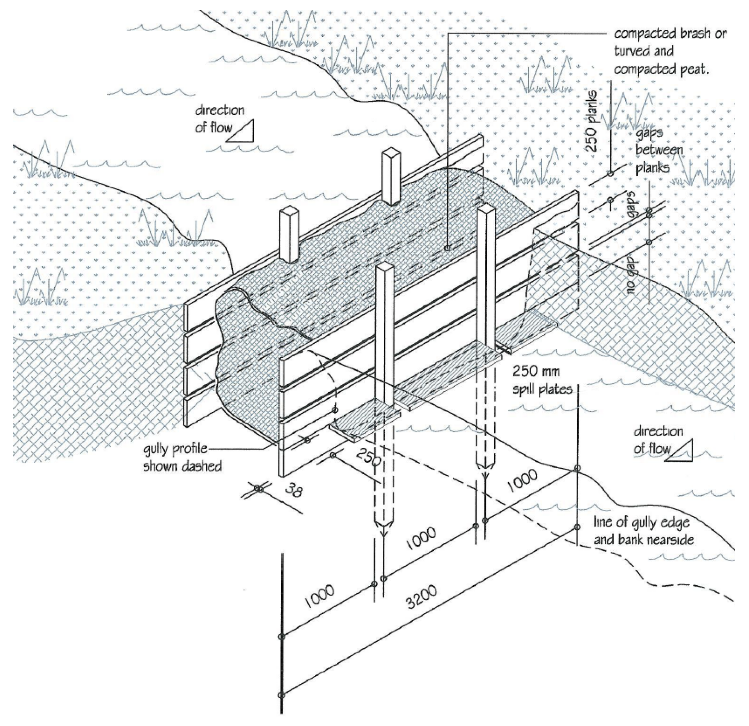
The installation technique is similar to that for plastic piling, with keying into basal material (peat or mineral substrate) and the ditch banks critical to success. Spill plates to control undercutting are also a requirement. As a significantly more substantial structure, their susceptibility to bowing and collapse is likely to be much less than either peat dams or plastic piling.
The following physical and hydrological changes to the bog may occur following restoration:
- The peat deposit may revert from a hydrologically and physically compartmentalised deposit to a more continuous hydrological unit; soil pipes crossing from one drain to another are unlikely to be blocked.
- The physical buttressing effect to upslope drain walls will be less than with peat dams but greater than for plastic piling (however composite dams are not often used extensively in individual drains due to their cost and complexity in installation).
- Water tables may rise in response to longer residence times of deeper water held between the dams (in the geomechanical approach, h will tend closer to unity, although in deeper peats the rise in water table will likely be a smaller proportion of the overall peat depth).
- There may be an associated increase in mass (due to saturation).
- There is unlikely to be any beneficial change in tensile strength at the bog surface.
Restoration effect scores are summarised in Table 4.21 below:
Table 4.21. Effects of wooden and composite dams on peat stability scores
Restoration effect |
Drain orientation |
Score |
Justification |
---|---|---|---|
Slope buttressing |
Downslope |
0 |
No buttressing due to drain alignment |
Slope buttressing |
Oblique / contour |
0 |
No buttressing due to large spacing |
Tensile strength |
Downslope |
-1 |
Close spacing allows binding |
Tensile strength |
Oblique / contour |
0 |
Larger spacing minimises binding |
Water table / pore pressure |
Downslope |
+1 |
Water table rise expected |
Water table / pore pressure |
Oblique / contour |
+1 |
Water table rise expected |
Water transmission in peat pipes |
Downslope |
0 |
No pipe blocking due to drain alignment |
Water transmission in peat pipes |
Oblique / contour |
0 |
No pipe blocking assumed due to large dam spacing |
Summary: for downslope aligned drains, there will be no net destabilising effect from installation of peat dams, while for drains oblique to slope or contour-aligned there will be a net destabilising effect (a score of +1).
4.4.1.4. Ditch Blocking - Reprofiling / Wave Dams / Zipping
Ditch reprofiling involves considerably more groundwork but is becoming a widely used technique as the number of skilled contractors increases. The technique involves redistributing peat from drain sides into the drain floor and better distributing surface vegetation (turves) across the former drain surface (see Figure 4.5). It is typically employed on relatively gentle slopes alongside intermittent drain blocking with dams (peat or other). Wave dams are similar in terms of the proportion of the drain reworked and brought into the drain alignment, though result in ponding between dams. Zipping is a further development of wave dams, with the drain sections between wave dams largely infilled by pivoting in blocks of peat from the drain sides to interlock (or ‘zip’) the void formed by the drain.
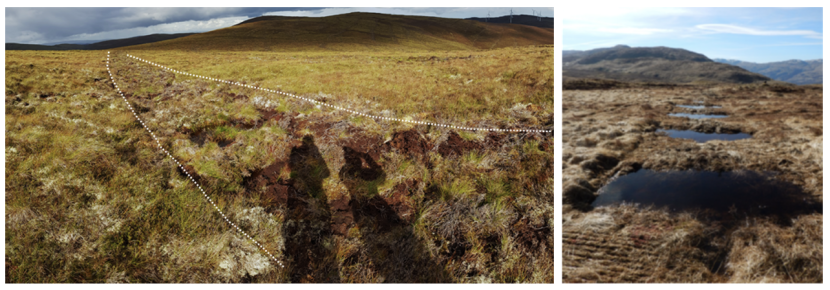
Types of drain reprofiling. Left: reprofiled ditch in blanket bog (former ditch alignment highlighted between hashed lines as visible as subtle difference in vegetation colour); right: wave dams.
Following restoration, changes to the bog are likely to be similar to those for peat dams:
- The peat deposit may revert from a hydrologically and physically compartmentalised deposit to a more continuous hydrological unit, although there is a high potential for soil pipes crossing from one drain to another to become blocked by the peat lining the ditches (see Figure 3.11).
- The infilling material will act as plugs of peat material buttressing the upslope drain walls.
- Water tables may rise in response to longer residence times of deeper water held between the dams (in the geomechanical approach, h will tend closer to unity, although in deeper peats the rise in water table will likely be a smaller proportion of the overall peat depth).
- There may be an associated increase in mass (due to saturation).
- The tensile strength of the acrotelm may increase across the peat deposit as a whole as transplanted turves knit together across the former drain alignment.
- There will be little change in geotechnical properties of the basal peat, since it is primarily the near surface peat layers that benefit from restoration.
It is important to note that the hydrological effectiveness and buttressing effect of reprofiling / zipping may be limited if the ‘spoil’ excavated from the original drain is replaced within it (as this peat is normally irreversibly damaged from drying above the ground surface).
Restoration effect scores are summarised in Table 4.22 below:
Table 4.22. Effects of reprofiling / wave dams / zipping on peat stability scores
Restoration effect |
Drain orientation |
Score |
Justification |
---|---|---|---|
Slope buttressing |
Downslope |
0 |
No buttressing due to drain alignment |
Slope buttressing |
Oblique / contour |
-1 |
Semi-continuous buttressing from peat plugs |
Tensile strength |
Downslope |
-1 |
Close spacing and semi-continuous turfs allow binding |
Tensile strength |
Oblique / contour |
-1 |
Larger spacing minimises binding |
Water table / pore pressure |
Downslope |
+1 |
Water table rise expected |
Water table / pore pressure |
Oblique / contour |
+1 |
Water table rise expected |
Water transmission in peat pipes |
Downslope |
0 |
No pipe blocking due to drain alignment |
Water transmission in peat pipes |
Oblique / contour |
+2 |
High likelihood of pipe blocking |
Summary: for downslope aligned drains, there will be no net destabilising effect from reprofiling / installation of wave dams / zipping, while for drains oblique to slope or contour-aligned there will be a net destabilising effect (a score of +1).
4.4.1.5. Ditches in raised bogs
For drains in raised bog settings where the ground surface is either flat or very gently sloping, the previously described approach to assessing impacts from ‘downslope’ and ‘oblique’ and ‘contour-aligned’ drains does not apply. Instead, the effects of each ditch blocking technique are considered to be largely the same and dependent upon the arrangement of drains in the raised bog as a whole (as detailed in Table 4.13). The principles are described below.
Buttressing effects only apply in shallow raised bogs <2.0m in depth and where there are peripheral drains in which a buttress might support the ‘enclosed’ dome behind it. A minor destabilising effect (+1) applies. This reflects the assumed negligible benefits from potential buttressing in the bog centre, where the bog will be at its ‘flattest’ and there will be minimal peat ‘upslope’ to buttress. The 2.0m threshold has been selected since in deeper bogs, the proportion of the peat mass that will be buttressed by the blocking of shallower drains is likely to be minor.
Water table recovery and associated increase in basal pore water pressures, is anticipated to slightly reduce stability for all peat drainage classes (a minor destabilising effect of +1).
Pipes have rarely been documented in raised bogs, and the relative lack in elevation change over a flat slope would preclude significant hydrostatic pressures building (from one end of a pipe to the other). Therefore pipe blocking effects are excluded from the assessment (no modifying score).
No other effects are considered
4.4.2. Bunding
Bunding comprises a range of techniques with two aims: i) to prevent water loss through cracks and pipes within the peat mass, and ii) to retain water on a bog surface by preventing ‘excess’ surface overland flow. Bunding is typically used in lowland raised bogs, but bunds of coir matting, turf, heather and netting have also been used in gullies in upland blanket bogs.
4.4.2.1. Trench / Backfill Bunding
Trench bunding is primarily recommended where surface dams are ineffective at the edges of lowland raised bogs (due to subsurface cracking at the dewatered raised bog margins). A trench is excavated below the anticipated crack depth and then infilled with peat, which due to bulking up is typically elevated above ground surface (usually up to c. 0.3m, see Figure 4.6). If ineffective (e.g. because the trench is too shallow), they may be bypassed. If they are too effective, there may be a considerable build up of water behind the bund (which ultimately may lead to breach). Both lowland raised bog case studies in which failures were observed involved bunding (see Section 3.2), although it is unclear to what extent the bunds contributed to failure. The Peatland ACTION technical compendium (SNH, 2019) recommends setting bunds at least 20m back from raised bog margins, but notes that many English bogs have bunds within 1m of their margins.
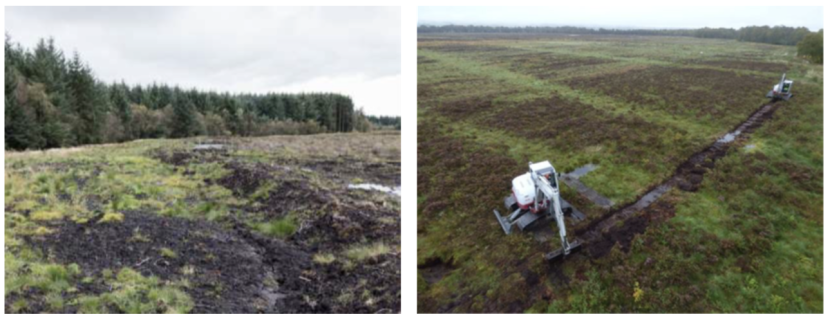
Following restoration using trench bunding, the following hydrological and physical changes to the bog may occur:
- Lateral subsurface flow of water within the acrotelm and upper catotelm may be impeded by the trenched material.
- Saturated excess overland flow of water at the bog surface may be impeded by the surface bund.
- The tensile strength of the surface peat will be lost along the bund alignment.
- Water content and mass of the peat will increase.
Noting that raised bogs typically fail as bog bursts (rather than peat slides) and that the failure surface is normally deep within moderate to deep peat (typically 3m-4m deeper or greater), it is unlikely that the hydrological impacts of bunding will drive instability (as the retaining / blocking effect of bunding will occur above potential failure surfaces). Equally the mass of water sitting on top of the bog will be minimal in comparison to the mass of underlying peat.
Dependent on the proximity of the bund and excavators to the bog margin, the loss of tensile strength and load effects from the excavators may be significant, however, this will be most keenly felt at the time of groundworks rather than afterwards.
The most likely form of ‘failure’ (other than margin collapse) is failure of the bund structure, releasing impounded water and incorporating the bund materials within the outflow to produce a peaty slurry. These effects may still be significant, but not as significant as a true large-scale bog burst
Restoration effect scores are summarised in Table 4.23 below:
Table 4.23. Effects of trenching / backfill bunding on peat stability scores
Restoration effect |
Drain orientation |
Score |
Justification |
---|---|---|---|
Slope buttressing |
Downslope |
n/a |
Trench bunding is generally applied along contour only |
Slope buttressing |
Oblique / contour |
0 |
Minimal change to buttressing from trench bunding |
Tensile strength |
Downslope |
n/a |
n/a |
Tensile strength |
Oblique / contour |
+1 |
Disruption of acrotelm by trenching reduces tensile strength |
Water table / pore pressure |
Downslope |
n/a |
n/a |
Water table / pore pressure |
Oblique / contour |
+1 |
Water table rise expected |
Water transmission in peat pipes |
Downslope |
n/a |
n/a |
Water transmission in peat pipes |
Oblique / contour |
0 |
Pipes unlikely to be blocked as bunding only affects shallowest part of soil profile |
Summary: for drains oblique to slope or contour-aligned there will be a net destabilising effect (a score of +2).
4.4.2.2. Bunding - Cell Bunding / Surface Bunding
Both cell bunding and surface bunding are variations on a similar technique, the purpose of which is to retain surface water. Surface bunds may be long linear features, and where connected by other orthogonal bunds may be referred to as cell bunds. These techniques do not involve disturbance to the underlying peat, though may involve some loss of tensile strength in the areas from which peat is extracted to build the bund. Examples of surface bunding are shown in Figure 4.7.
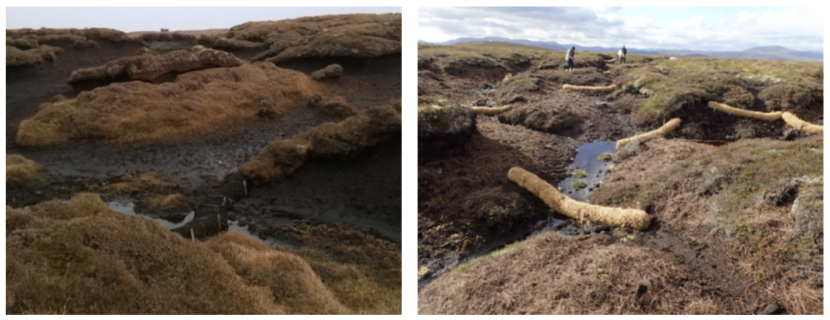
Following restoration using surface bunding (including cell bunding), the following hydrological and physical changes to the bog may occur:
- Saturated excess overland flow of water at the bog surface may be impeded by the surface bund.
- Water content and mass of the peat may increase.
Since the effects of cell bunding and surface bunding are almost exclusively on the very shallow surface hydrological regime of the bog, with little or no subsurface hydrological or physical effects on the peat mass, these techniques are not expected to affect the susceptibility of blanket or raised bogs to instability.
Summary: no impact on peat stability.
4.4.3. Gullies
Gully restoration has been long practiced in the UK, with significant research into methods undertaken by the Yorkshire Peat Partnership (YPP) and the Moors For The Future Partnership. A series of technical notes were prepared by YPP in 2010 and revised in 2014 and 2017.
Gully restoration techniques proposed by Peatland ACTION utilise similar techniques to those for ditches, but on a larger scale. Unlike drained peatlands, gullied peatlands, in particular those that are heavily eroded, are significantly more hydrologically discontinuous than areas of blanket peat subject to drainage (see Figure 2.12 and Figure 4.8). They will have been more extensively dewatered due to the usually high drainage density of gully networks and the extent of exposed bare peat that comprises gully sidewalls. Gullies, both dendritic and linear, tend to align downslope rather than obliquely or along contour and are therefore not as effective in removing toe support as drains. Gully formation also tends to be progressive rather than sudden, giving the peat time to hydrologically and physically adapt.
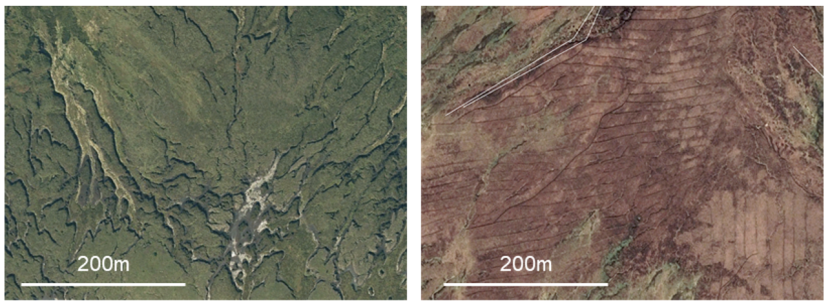
Given this, gully restoration techniques are not expected to negatively impact peat instability at a scale equivalent to peat slides and bog bursts. Instead, instability should it occur is more likely to be minor in scale and associated with failure of the within gully works.
4.4.3.1. Gullies - Reprofiling
Gully reprofiling operates on similar principles to ditch reprofiling by reducing the slope of the gully sidewalls and relaying peat turves (which often hang over gully walls) to better protect the reworked peat. This may involve breaking turves up and redistributing them, and re-seeding or geotextile may also be used to provide additional protection. Where gullies are wide, reprofiling may not provide continuous peat cover from one side of the gully and damming or bunding may be required (see below).
During restoration, the primary instability risk will relate to the effect of the excavator when working above the gully sidewall (top and bottom working are required).
Subsequent to restoration, the primary effect of reprofiling will be to reduce the likelihood of small scale instability of the sidewalls (e.g. Figure 2.3a) and increase toe support to the gully sides. Therefore, restoration is considered to have a beneficial effect on peat stability, provided excavator operators follow good practice and safe methods of working when above gully sides.
Restoration effect scores are summarised in Table 4.24 below:
Given this, gully restoration techniques are not expected to negatively impact peat instability at a scale equivalent to peat slides and bog bursts. Instead, instability should it occur is more likely to be minor in scale and associated with failure of the within gully works.
Table 4.24. Effects of gully reprofiling on peat stability scores
Restoration effect |
Score |
Justification |
---|---|---|
Slope buttressing |
0 |
Reprofiling does not introduce sufficient material for buttressing and gullies are usually aligned downslope |
Tensile strength |
0 |
Spreading out of turves to cover bare faces unlikely to increase binding in short term |
Water table / pore pressure |
0 |
Primary effect of reprofiling is to reduce bare peat cover rather than significantly raise water tables |
Water transmission in peat pipes |
0 |
No pipe blocking due to gully alignment |
Reduction in slope severity |
-1 |
Local reduction in steep slopes reduces potential small scale slippage that might otherwise trigger larger-scale peat failure |
Summary: there will be a minor stabilising effect on peat arising from the reduction in the extent of locally steep slopes (-1 to likelihood).
4.4.3.2. Gullies - Damming / Bunding
Damming and bunding may be undertaken using a range of materials, typically stone or composite dams (e.g. wood and peat). Dams or bunds are generally designed to be ‘leaky’, i.e. to prevent build-up of excess water behind the dams to a point where the structures could fail and release retained material down the gully axis.
Subsequent to restoration, the primary effect of damming and bunding would be to hold more water within the gully floor, encourage sedimentation (of washed peat and mineral soils) and provide wet conditions within which peat forming species may prosper. Provided that restoration follows good practice in relation to maintaining leaky dams, damming and bunding are not expected to have a detrimental effect on peat stability.
Restoration effect scores are summarised in Table 4.25 below:
Table 4.25. Effects of gully damming / bunding on peat stability scores
Restoration effect |
Score |
Justification |
---|---|---|
Slope buttressing |
0 |
Reprofiling does not introduce sufficient material for buttressing and gullies are usually aligned downslope |
Tensile strength |
0 |
Distribution of turves to cover bare faces (e.g. ‘chess-boarding’) unlikely to increase binding in short term |
Water table / pore pressure |
0 |
Primary effect of reprofiling is to reduce bare peat cover rather than lead to significant water table recovery |
Water transmission in peat pipes |
0 |
No pipe blocking due to gully alignment |
Reduction in slope severity |
0 |
No reduction in steep slopes so local instability remains possible (though no more than previously) |
Summary: there will be no impact on peat stability.
4.4.4. Bare Peat (revegetation, spot-turving and transplanting)
While applicable to the bare peat within gully systems, bare peat can also be present due to dewatering of pool and hummock systems and where wind erosion (deflation) dries, breaks up and removes exposed surface peat. Revegetation techniques, including spot-turving and transplanting have minimal physical impact on the peat and are not considered further here, however, in the long term once translocated turves have bound into their peat substrate and extended laterally to join one another, there will likely be beneficial tensile effects.
Summary: no (short term) impact on peat stability.
4.4.5. Forest to Bog restoration
Forest to bog restoration involves the reversal of afforestation to return blanket or raised bogs to open bog habitat. Due to the extent of planting in Scotland (and elsewhere), considerable efforts have been made to investigate the effectiveness of forest to bog restoration. FCE and latterly FLS have numerous large-scale trial plots within which a range of techniques are being tested. Typically, forest to bog restoration involves various combinations of:
- Tree felling and timber removal.
- Ditch and furrow blocking.
- Mulching.
- Ground smoothing/stump flipping.
Of these techniques, mulching is unlikely to significantly impact either the hydrology or physical characteristics of peat (other than in reducing drying), as it effectively top dresses the formerly afforested peat surface, infilling furrows and ditches (therefore the loads are relatively small and there is little or no disturbance to the subsurface). The other three techniques are considered below.
4.4.5.1. Forest to Bog - Tree Removal
The first stage of restoration is tree removal, usually when timber is commercially viable or alternatively if the site is redesignated for restoration. “Tree” removal can refer to both the harvestable stems (trunks) and to the branches (brash). While brash has been left on some sites as brash mats in order to facilitate passage of harvesting plant, the increasing use of low ground pressure machines has reduced this requirement. It is generally preferable to remove brash from site rather than leave it on the ground as it can make restoration work difficult and can suppress growth of bog vegetation. The only component of the tree left in place is the stump (cut as low to ground level as possible) and the subsurface root plate within the ridge line.
On its own, tree removal does not constitute restoration, although avoiding restocking and removal of natural regeneration would imply some degree of intent to restore a formerly afforested peatland. The physical and hydrological effects of tree removal are as follows:
- Water table rebound (rise) due to lack of uptake by the now absent tree stock; typically, post afforestation, the water table lies below the base of the furrows between ridges (see Figure 4.9).
- Reduction in destabilising load due to removal of trees is countered by decrease in mass of peat from risen water table and reduction in contact pressure between the peat and substrate which reduces the friction.
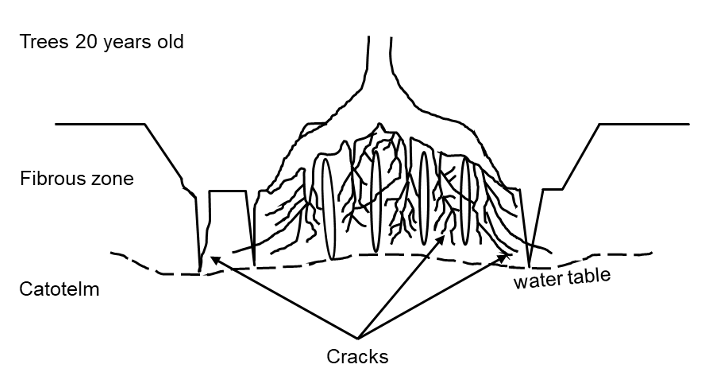
Restoration effect scores are summarised in Table 4.26 below:
Table 4.26. Effects of tree removal on peat stability scores
Restoration effect |
Score |
Justification |
---|---|---|
Slope buttressing |
0 |
n/a |
Tensile strength |
0 |
Tensile effects of root plates remains as trees are harvested above ground level |
Water table / pore pressure |
+1 |
Water table rise expected |
Water transmission in peat pipes |
0 |
n/a (laterally continuous pipe networks unlikely to be present due to disruption of peat hydrology) |
Loading |
0 |
Removal of trees reduces gravitational load but also reduces friction at the base of the peat |
Increasingly, tree removal is just the first step in restoration and the effects of additional restoration groundworks are described below.
Summary: in isolation, tree removal results in a net destabilising effect (a score of +1).
4.4.5.2. Forest to Bog - Drain Blocking
Forest drains are often cut oblique to slope and parallel to ridges and furrows or as perimeter drains around individual stands of trees. The effect of drainage is similar to that for unforested blanket bogs, as are the effects of their restoration. The use of brash and woody debris for ditch blocking is more frequent in afforested peatlands, not least because driving plastic piling into peat with ample tree roots can be difficult, as can reprofiling or blocking with peat dams (for the same reasons).
The effects of ditch blocking are greater than furrow blocking in terms of the physical and hydrological properties of the peat, and are considered to be as for the various forms of ditch blocking described in Section 4.4.1.
4.4.5.3. Forest to Bog - Ridge and Furrow Blocking
Ridge and furrows are formed during ground preparation for planting and have a number of effects on peat soils, including disruption of the vegetated acrotelm (lowering tensile strength) and disruption of the surface hydrology within the acrotelm. In some cases, brash or mulch may be placed along the furrows to smooth the ground surface, sometimes in combination with peat dams.
Restoration effect scores are summarised in Table 4.27 below:
Table 4.27. Effects of ridge and furrow blocking on peat stability scores
Restoration effect |
Score |
Justification |
---|---|---|
Slope buttressing |
0 |
Minimal effect due to shallow depth of furrows |
Tensile strength |
0 |
Minimal effect as brash or mulch does not necessarily encourage revegetation, root plates remain in situ |
Water table / pore pressure |
0 |
No additional effect above that imparted by tree felling (considered above) |
Water transmission in peat pipes |
0 |
n/a |
Loading |
0 |
No additional effect above that imparted by tree felling (considered above) |
Summary: ditch (and furrow) blocking has no effect on peat stability.
4.4.5.4. Forest to Bog - Ground Smoothing / Stump Flipping
Ground smoothing following felling aims to remove the ridges and furrows created by double mouldboard ploughs in order to encourage more equal distribution of water at the bog surface and avoid leaving the ridges to dry further above the ‘average’ ground surface. Ditch blocking can be a by-product of this restoration approach.
Stump flipping is typically the first stage in ground smoothing, in which an excavator bucket is used to turn the stump and underlying root plate over into the adjacent furrow. The inverted woody mass is then driven into the peat surface with the bucket. Once enough stumps have been flipped, the area is cross tracked, further driving the woody debris into the peat and producing a largely level and usually bare peat surface (see Figure 3.17).
The amount of woody debris incorporated into the peat usually depends on the condition of the trees and/or the success of the timber harvest. In areas of extensive windthrow, trees may not be harvested, and the stems, stumps and brash may all be incorporated into the underlying peat. At the other extreme, only the stumps may be left and these are all that is incorporated. Modelling the geomechanical effects of these techniques on the peat is considered to be overly subjective. However, the primary effect of incorporation of woody debris is likely to be an increase in tensile strength of the peat mass through interlocking of woody material. The greater the amount of material incorporated, the greater the benefit. There may also be an effect from the creation of void spaces within the peat in small pockets between woody material. These voids were visible near surface during walkover of the FLS trial sites. Although ground pressure from excavators has the potential to increase pore pressures (over very short periods as the excavator passes), the presence of numerous voids around woody material would, in likelihood, allow water to be redistributed through voids and prevent this increase occurring.
Restoration effect scores are summarised in Table 4.28 below. The table primarily considers the varying tensile effects of different combinations of stump and brash treatment. The effects of cross tracking of plant is considered to be negligible (due to the very transient nature of the loads) and water table effects are considered to be negligible in comparison to those from tree removal (which is covered above).
Table 4.28. Effects of ground smoothing / stump flipping on peat stability scores
Felling waste treatment |
Score |
Justification |
---|---|---|
Stump flipping |
0 |
Minimal effect due to shallow depth of furrows |
Stump flipping + brash incorporation |
-1 |
Minimal effect as brash or mulch does not necessarily encourage revegetation, root plates remain in situ |
Stump flipping + brash + stem incorporation |
-2 |
No additional effect above that imparted by tree felling (considered above) |
Summary: overall, while deforestation without restoration has a minor destabilising effect, any proactive restoration works (such as ditch blocking, woody debris incorporation or cross-tracking) will have either a neutral or stabilising effect dependent on the chosen technique.
4.4.6. Scrub Removal
Scrub removal involves returning bog to open habitat by hand removal of scrub or cutting to ground level. Groundworks are minimally intrusive / negligible and there will be minimal physical change to the peat. In the short to medium term, the drawdown of the water table by shrubs will be reduced, but this raise in water table is expected to have minimal effect.
Summary: no impact on peat stability.
5. A risk-based protocol for assessing proposed peatland action restoration sites
5.1. Defining peat landslide risk
It is generally accepted that risk is the potential for adverse consequences, loss, harm or detriment (Scottish Government, 2017). Peat landslides are a form of geohazard, i.e. a geological or geomorphological process that in particular circumstances could lead to loss or harm.
Typically, when considering landslides, risk is expressed as the product of the likelihood of a landslide event and its adverse consequences (after Lee and Jones, 2013):
Risk = Likelihood of Peat Landslide × Adverse Consequences
The likelihood of a peat landslide occurring or the susceptibility of a site to the occurrence of a peat landslide reflects the site conditions and triggering factors. Site conditions may include peat depth, slope angle, land use and hydrology, while triggering conditions may be natural, e.g. heavy rainfall, snowmelt, or human induced e.g. cutting or loading of slopes. Baseline site conditions and their influence on susceptibility were considered in Section 4.2 of this report while restoration groundworks were considered as triggers in Section 4.4.
Adverse consequences may include accidents, loss of life, environmental impacts or damage to infrastructure (such as roads) and associated financial losses. The potential for adverse consequences reflects the exposure to peat landslide hazard of elements at risk within a specific area. For example, a designated watercourse at the foot of a hill may always be exposed to the effects of a peat landslide, but walkers using hill paths may only occasionally be exposed (when using the paths).
5.2. Objectives of the risk-based protocol
The objective of the risk-based protocols attached to this report are to enable Peatland ACTION officers to identify the potential impacts of restoration activities on peat stability and facilitate decision making on the suitability of restoration activities according to site conditions, existing technical knowledge of peat instability as a phenomenon, and considering the full range of benefits and potential risks associated with restoration activities. At the same time, it is recognised that Peatland ACTION officers are not geotechnical specialists and that decision making as to the suitability of restoration techniques is to be undertaken at a site-scale.
There are various levels of risk assessment, ranging from high-level qualitative assessments where the objective is to develop an approximate estimate of the risks (e.g. low, medium, high) and more detailed quantitative risk assessments (QRA) where the objective is to generate more precise measures of the risks (e.g. expressing risk as a specific magnitude of loss). For the scale of decision making required for restoration sites, a high-level qualitative approach is the most appropriate.
Two risk-based protocols have been generated, one for blanket bogs and one for raised bogs, reflecting their different settings and physical conditions (see Section 2.3.3). The protocols have been trialled with Peatland ACTION officers at workshops prior to completion of this report and were agreed to provide a usable level of detail for practitioners without a geoscience background. The following sections describe the structure and usage of each protocol. Annex 3 provide accompanying ‘guidance notes’ for each protocol and provide further information on their usage.
5.3. Structure of the risk-based protocols
The blanket bog and raised bog protocols have been provided as Microsoft Excel workbooks comprising five worksheets. Each worksheet requires inputs from Peatland ACTION officers based on site information and proposed restoration practices in order to progress the risk assessment process. The five worksheets and their functions are as follows:
- Front Sheet: summary text describing the purpose of the workbook and outlining the steps required by Peatland ACTION officers to implement the protocol.
- Step 1. Pre-Restoration Likelihood: a series of questions and answers used to determine the likelihood of a peat landslide under natural (baseline) conditions.
- Step 2. Post-restoration Likelihood: a series of questions and answers used to determine the impact of proposed restoration techniques on the baseline likelihood (from Step 1).
- Step 3. Consequence and Risk: dependent on the likelihood of instability (from Step 2), if required, a series of questions and answers used to identify potential consequences and associated risks for 'receptors' within and adjacent to the site.
- Summary Form: a one-page summary sheet of inputs and outputs from the protocol to be generated as a summary of the protocol findings.
This process is shown schematically in Figure 5.1. The protocols determine the ‘baseline’ or unmodified likelihood of a peat landslide and then the potential impacts or ‘modified’ likelihood associated with restoration works. If the likelihood of a landslide is low enough (such that risks of Medium or higher cannot be generated), then restoration may proceed.
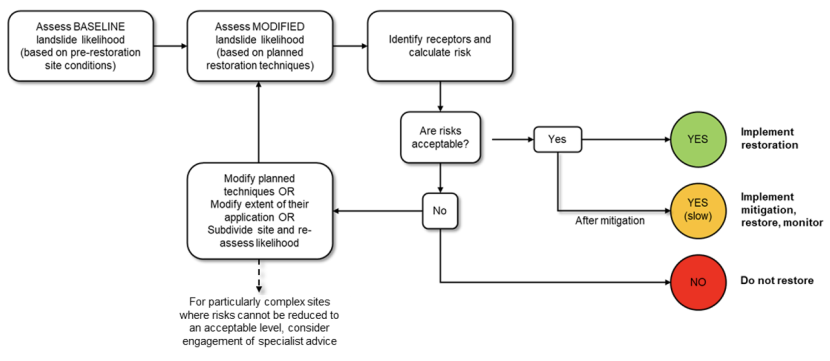
If restoration works generate Moderate or higher likelihoods, then there may be potential for Medium or higher risks and either the proposed measures must be reviewed (to reduce the likelihood, and, in turn, risk) or the site must be partitioned into smaller units to identify which parts of the site have lower likelihoods of instability and therefore may be more acceptable to restore.
Sections 5.3.1 to 5.3.3 summarise the content of each worksheet (with full details provided in Annex 4). Figure 5.2 shows excerpts of the worksheets for context.
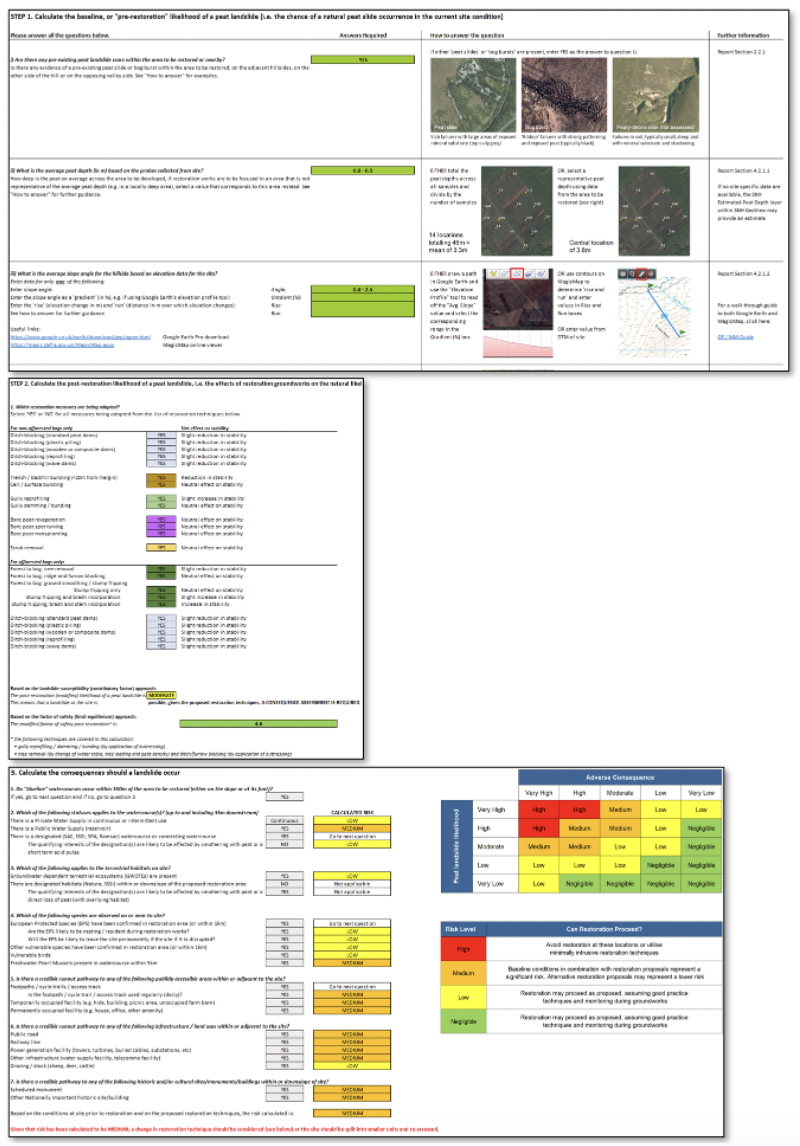
5.4. Using the risk-based protocols
The blanket bog and raised bog risk-based protocols comprise standalone Microsoft Excel Workbooks and are provided as electronic appendices to this report. The protocols have identical structures, with minor differences in the inputs required to calculate risk. The following sections describe how to use the protocols to calculate risk.
5.4.1. Determining baseline stability (or likelihood of a landslide)
Step 1 determines the baseline likelihood through a combination of qualitative and geomechanical (Factor of Safety) approaches and using a series of questions with pre-defined answers, the most appropriate of which are selected for the site in question by the Peatland ACTION officer. The baseline likelihood is calculated as a numerical score (out of 24) based on the eight factors scored from 0 to 3 and described in Sections 4.2.1 (blanket bog) and 4.2.2 (raised bog). Likelihood classes from Very Low to Very High are based on the scores shown on Table 4.9 and Table 4.15.
Table 5.1 shows the questions and answer format (either as a drop-down selection or as a site derived input). Outline guidance on completing the questions is provided in a “How to answer the question” column on the protocol with additional guidance in the accompanying guidance note (reproduced in Annex 3).
Table 5.1. Questions used to determine baseline peat landslide likelihood
Question |
Answer format |
---|---|
Q1 Are there any pre-existing peat landslide scars within the area to be restored or nearby? |
Dropdown choice, either YES or NO. |
Q2 What is the average peat depth (in m) based on the probes collected from site? |
Dropdown choice, site specific peat depth data are required |
Q3 What is the average slope angle for the hillside based on elevation data for the site? |
Dropdown choice, slopes are derived from GIS layers available to NS |
Q4 What is the substrate? |
Dropdown choice, substrate is derived from freely available BGS data or from site observations |
Q5 Which of the following best describes the geomorphology of the peat on site? |
Dropdown choice based on visual legend of landforms compared with review of Google Earth ProTM |
Q6 Are any of the following land uses present over the area to be restored? |
Dropdown choice based on review of Google Earth ProTM or site visit |
Q7 Which of the following best describes the status and condition of forestry on site? |
Dropdown choice based on comparison of forestry row alignment with hillslope contours (based on review of MagicMap and Google Earth ProTM) |
Q8 Which of the following best describes the slope profile of the area to be restored? |
Dropdown choice based on profile tool in GoogleEarthTM or review of contours on MagicMap |
Q9 Which of the following best describes artificial drainage in the area to be restored |
Dropdown choice based on comparison of drain alignment with contours (based on review of MagicMap and Google Earth ProTM) |
Q10 What type of peat is found at the base of the area to be restored |
Dropdown choice based on site specific peat observations – an optional input that is only required to generate a Factor of Safety output |
The results of the baseline assessment are reported as follows:
- A baseline landslide likelihood class (one of Very Low, Low, Moderate, High or Very High).
- A written statement explaining in non-technical language what this likelihood class means.
- A statement of the most likely form of peat instability (either ‘peat slide’ or ‘bog burst’, or if likelihood is Low or Very Low, ‘minor instability’).
- Where Q10 has been answered, a Factor of Safety result.
At the end of Step 1, the baseline landslide likelihood class and site averaged Factor of Safety should be recorded on the Summary Form. These ‘static’ results can then be compared with the modified results if mitigation is required following specification of restoration techniques and input of site receptors.
The next stage of the process is Step 2, in which proposed restoration groundworks are identified and the ‘modified’ post-restoration peat landslide likelihood is calculated.
5.4.2. Post-restoration landslide likelihood
Step 2 requires the user to select either ‘YES’ or ‘NO’ using a series of drop-down boxes to identify which restoration techniques will be used on the site. The list of techniques is separated into bogs that have not been subject to forestry and afforested bogs, and is as follows:
For non-afforested bogs only:
- Ditch-blocking (peat dams)
- Ditch-blocking (plastic piling dams)
- Ditch-blocking (wooden or composite dams)
- Ditch-blocking (dain reprofiling)
- Ditch-blocking (wave dams)
- Ditch-blocking (zipping)
- Deep bunding (trench / backfill)
- Cell / surface / fish-scale / scallop / finger bunding
- Gully reprofiling
- Gully damming / bunding (stone / surface bunds)
- Bare peat textile application
- Bare peat whole turving
- Bare peat transplanting, seeding or mulching
- Scrub removal
For afforested bogs only:
- Forest to bog: tree harvesting
- Forest to bog: ditch and furrow blocking
- Forest to bog: ground smoothing / stump flipping
- Stump flipping only
- Stump flipping and brash incorporation
- Stump flipping, brash and stem incorporation
- Ditch-blocking (peat dams)
- Ditch-blocking (plastic piling dams)
- Ditch-blocking (wooden or composite dams)
- Ditch-blocking (drain reprofiling)
- Ditch-blocking (wave dams and/or zipping)
The effects of each restoration technique on the baseline landslide likelihood are based on modifiers to the summed likelihood from Step 1 in line with the descriptions of restoration effects in Section 4.4. Within any cluster of techniques (e.g. the ditch-blocking techniques), the worst case modifier is extracted such that combination effects are only considered across different groups of techniques. For example, if two types of ditch blocking are specified in combination with one type of bunding, the most destabilising effect of the two ditch techniques will be combined with the effect of bunding to calculate the ‘modified’ likelihood score.
As well as reporting the net effect on stability of each selected restoration technique, a modified summed likelihood is generated and translated into a landslide likelihood class (Very Low, Low, Moderate, High, Very High). A revised Factor of Safety is also calculated based on the geomechanical effects of the restoration techniques (see 4.3.3).
At the end of Step 2, the post-restoration (modified) landslide likelihood class and site averaged modified Factor of Safety should be recorded on the Summary Form.
Where the ‘modified’ likelihood is Moderate or greater, the protocol indicates that “a consequence assessment is required”. If a Very Low or Low likelihood is calculated, restoration may proceed as planned without the need for a consequence assessment.
The consequence assessment and calculation of risk are implemented in Step 3.
5.4.3. Identifying receptors and consequences
Step 3 considers the full range of receptors that might be exposed to peat landslide hazards in both blanket peat uplands and areas with lowland raised bogs. In the uplands, which are typically more remote, these relate primarily to ecological receptors (such as watercourses, terrestrial habitats and species) whilst in lowlands, the public and/or public infrastructure may also be located relatively close to raised bogs. The list of receptors was agreed with the Peatland ACTION team during development of the protocols and is as follows:
Watercourse-related receptors:
- Un-designated watercourses / waterbodies without record of water supply within 5km.
- Watercourse with a private water supply (within 5km).
- Watercourse with a public water supply (within 5km).
- Designated watercourse / waterbody (SAC, SSSI, Ramsar within 5km).
Terrestrial habitats:
- No GWDTE present, no designation.
- No designation, but groundwater dependent terrestrial ecosystems (GWDTEs) present
- Designated site (SAC, SSSI).
Species:
- Vulnerable EPS (otter) confirmed in restoration area (within 1km).
- Other vulnerable species (water vole, water shrew, badger, adder) confirmed in restoration area.
- Vulnerable ground nesting birds – Schedule 1 or Red- listed ground nesting birds confirmed in the restoration footprint (e.g. black-throated diver, common scoter, hen harrier, red-throated diver, merlin). These species only lay one clutch of eggs per year and a loss of a breeding season could have a negative impact at a population level, (for scoter and black-throats in particular).
- Freshwater pearl mussels (FWPM) confirmed within or near restoration area (5km).
Publicly accessible areas:
- Footpaths / cycle trails / access tracks.
- Temporarily occupied facility (e.g. hide, building, picnic area, unoccupied farm barn).
- Permanently occupied facility (e.g. house, office, other amenity).
Infrastructure / land use:
- Grazing (e.g. sheep, cattle, deer).
- Arable (standing crops, productive land).
- Public highway / minor road.
- Other infrastructure (e.g. water supply facility, telecoms facility).
- Railway line.
- Power generation facility (e.g. towers, turbines, buried cables, substations, and watercourse supporting hydro scheme).
Historic and Cultural Environment
- Nationally important historic features, buildings, artefacts (e.g. scheduled monument).
The severity levels have been informed by existing published NS approaches to valuing ecological and historical and cultural environment receptors in Scotland (Table 1; SNH, 2015b).
Following a calibration exercise undertaken after one year’s use of the protocols, the consequence assessment is structured around the credibility of impacts for each receptor type. For example, where nesting species have been observed at a proposed restoration site, the assessment requires input on the likelihood of the species being in residence at the time of restoration or their permanent displacement if the site is disrupted. Similarly, for infrastructure (such as railways), the assessment queries whether there is a credible runout pathway from the potential source (the restoration area). Guiding principles on runout are provided in section 5.4.4 below.
For watercourse receptors (such as freshwater pearl mussels or designated waterbodies) a distance of 5km has been selected as a conservative estimate of the downstream zone of influence of a peat landslide, based on peaty runout being buoyant and highly mobile. It is judged to be very unlikely for any significant volume and/or concentration of peaty debris to be present beyond this distance from the point of ingress into a watercourse. In most cases, downstream transmission of peaty runout would be significantly less.
For each of the receptors listed above, the user is required to enter either ‘YES’ or ‘NO’ in a series of dropdown boxes. Each receptor has a pre-determined qualitative severity level, listed in Table 5.2. Five categories of severity (Very Low, Low, Moderate, High, Very High) are used, with all consequences benchmarked against ‘Very High’ for loss of life or loss of national level infrastructure. In practice, no receptors are considered to have Very Low severity in the event of a landslide impact. While it is accepted that any negative outcome is undesirable, these benchmarks were agreed by the Peatland ACTION team to be the most appropriate upper categories.
At the end of Step 3, the top receptors should be recorded on the summary form alongside the initial calculated risks.
Table 5.2. Severity levels and descriptions for receptors
Watercourses, waterbodies and aquatic habitats
Receptor |
Description of consequences |
Severity level |
---|---|---|
Un-designated watercourses |
Although aquatic habitats are valuable, if undesignated there is an assumption of low consequence |
Low |
Watercourse with a private water supply |
Consequences for (localised) private supplies can be mitigated through temporary measures |
Moderate |
Watercourse with a public water supply |
Consequences for public water supplies are unlikely to be easily mitigated |
High |
Designated watercourse |
Damage to qualifying interests are assumed to be a worst case environmental impact |
High |
Terrestrial habitats
Receptor |
Description of consequences |
Severity level |
---|---|---|
No GWDTE or designated habitats |
Terrestrial habitats with no GWDTE or designation and requiring restoration are assumed to be of limited value |
Low |
Groundwater dependent terrestrial ecosystems |
Although a site may host GWDTE, the need for restoration implies a compromised habitat |
Moderate |
Designated site |
Damage to a designated terrestrial habitat (Natura 2000, SSSI) is assumed to be a worst case environmental impact |
High |
Species (where not included in an over-lapping designation)
Receptor |
Description of consequences |
Severity level |
---|---|---|
EPS confirmed in restoration area (or within 1km) |
No impact at population level but impacts to an individual could occur. Restoration work generally avoids these species, informed by survey so potential impact already partly mitigated. |
Low |
Other vulnerable species confirmed in restoration area (or within 1km) |
Includes potential impacts on water voles, water shrew, badgers, adders (and their hibernaculum) |
Low |
Vulnerable Birds |
Ground nesting Schedule 1 and Red listed species confirmed in restoration footprint area (or within 1km). They would only be affected during their breeding season. Restoration work itself avoids the breeding season where these species are confirmed, but a peat landslide wouldn’t necessarily take place during restoration groundworks. |
Moderate |
FWPM present in watercourse within 5km |
Impact at population level is possible. |
High |
Site users (within and adjoining site)
Receptor |
Description of consequences |
Severity level |
---|---|---|
Footpaths / cycle trails / access tracks |
Low exposure due to intermittent use / potentially high consequences if injury or loss of life |
High |
Temporarily occupied facility |
Low exposure due to intermittent use / potentially high consequences if injury or loss of life |
High |
Permanently occupied facility |
High exposure due to occupancy / potentially high consequences if injury or loss of life |
Very high |
Infrastructure / land use
Receptor |
Description of consequences |
Severity level |
---|---|---|
Grazing |
Consequences for local landowner, including loss of income and requirement to restock |
Moderate |
Arable |
Consequences for local landowner, including loss of crop/income and potential medium term impacts on land productivity |
High |
Public highway / minor road |
Easily repaired and cleared with low to high exposure (depending on use) and potentially high consequences if injury or loss of life |
High |
Other infrastructure (e.g. water supply facility, telecoms, other) |
Consequences for local, regional or national infrastructure are likely to be complex to repair |
High |
Railway line |
Complex to repair and clear, disruption to important routes, potentially high consequences if injury or loss of life |
Very high |
Power generation facility |
Complex to repair and clear, disruption to power supply (with associated indirect effects locally, regionally or nationally) |
Very high |
Historic and cultural environment
Receptor |
Description of consequences |
Severity level |
---|---|---|
Scheduled Monument or other Nationally important historic site/building on or downslope of restoration area |
Scheduled Monuments are considered to be of national importance and are subject to statutory protection. |
High |
5.4.4. Determining credible runout pathways
The consequence assessment requires the protocol user to make a series of decisions about the credibility of a landslide impact should a landslide occur. This decision requires an understanding of the typical scale of a landslide and the behaviour of a peat landslide once it has occurred. This varies depending on the landslide type.
For peat slides:
- The majority of peat slides partially or fully evacuate the scarp area and undergo runout downslope.
- The combined scarp and runout lengths are typically of the order of 250-500m, with c. 90% of peat slides being <500m in length from headscarp to the downslope limit of runout. The average total length is c. 250m.
- Peat slide debris rapidly thins with distance (peat blocks reduce in number and size).
Following calibration of the protocols after a year of use, the ‘Consequence and Risk’ sheet has been substantially reworked to guide users to consider these issues through the format of the questions. However, if further assistance is required, where the protocols indicate a ‘peat slide’ as the most likely form of instability, the user should consider the following:
- Where is the ‘receptor’ located relative to the area assessed in the protocol? If the receptor is >500m from the nearest restored feature, there is unlikely to be a credible pathway to the receptor. If the receptor is upslope of the nearest feature to be restored or is on an adjacent valley side, then there is unlikely to be a credible pathway. If the receptor is elevated relative to the ground surface over which the landslide runout would occur (e.g. a railway or road embankment), then there is unlikely to be a credible pathway.
- Are there physical barriers to impacts on the receptor? For example, although bog bursts triggered within afforested areas may mobilise tree cover, peat slides have not been recorded breaching tree lines. Similarly, substantial wall structures (e.g. stone walls) may impede runout, buried services may simply be passed over (peat lacks the density to eroded most surfaces), or in-channel retention structures (e.g. dams) may impede downstream transmission of debris.
- Are the vulnerable characteristics of the specific receptor likely to be affected? For designated aquatic and terrestrial habitats, are the qualifying interests likely to be affected? For example a river may hold a SAC designation, but the designation may relate to riverside habitats rather than the watercourse itself. For public access receptors, the footpath may be so infrequently used that there is minimal exposure of the public to risk.
The logic is similar for bog bursts:
- The majority of bog bursts only partially evacuate their ‘source zones’ (which can lack a clear headscarp).
- However, runout from the source zone is often similar in character and behaviour to that of peat slides.
- The combined length of the source and runout zone in bog bursts is generally greater than for peat slides with over 35% of failures >500m in length. The average total length is c. 430m.
When addressing the credibility of impacts from bog bursts, the considerations are largely as for peat slides above, with the following variations:
- Where is the ‘receptor’ located relative to the area assessed in the protocol? As retrogressive failures (see Section 2.2), failures may cut upslope from the point of initiation, and therefore receptors 100-200m upslope of the proposed restored features may also be at risk.
- Are there physical barriers to impacts on the receptor? Bog bursts have demonstrably mobilised afforested areas, and may extend outside forest margins into adjacent unforested areas (again, possibly 100-200m from a treeline).
Common sense should be applied when considering potential risks and the results of the assessment judged accordingly.
5.4.5. Calculating risk
The risk calculation process is carried out as part of Step 3, with risks calculated as a product of the post-restoration likelihood and the perceived consequence levels for each receptor listed in Section 5.4.3.
Calculated risks are shown for each receptor identified as present within or adjacent to the site and the highest risk outcome is identified. The protocol indicates whether restoration proposals should be reconsidered according to the calculated level of risk:
- If risks are Low or Negligible, restoration should proceed (in line with the process shown on Figure 5.1).
- If risks are Medium, restoration techniques should be reconsidered or the site split into smaller units and re-assessed (with the higher likelihood or higher consequence areas separated from the wider site).
- If risks are High, restoration should be avoided or reconsidered, e.g. through low impact techniques (such as bare peat revegetation, spot turving or transplanting.
A further ‘sense check’ on the ratio of proposed restoration to potential size of landslide has also been added to protocols in order to provide some measure of potential gains (from restoration) against potential losses (due to mobilisation and loss of embodied carbon within the peat displaced during the landslide). This step only applies to sites with a calculated Medium or High risk where a further consideration may be required to progress a proposal.
In summary:
- A series of worst case (conservative) volume losses were identified for a peat landslide, specific to half metre depth ranges used as site averages in the protocol – the deeper the peat, the larger the peat volume loss (from c. 35,000m3 for 0.5m depth to >200,000m3 for >3.0m depth).
- A worst case water table drawdown effect was calculated for each landslide ‘size’ (based on a credible area associated with each volume) equivalent to draining the top metre of a 30m zone extending around the scarp / source zone for each landslide size – peat within this zone was considered an additional ‘volume loss’.
- A metric of ‘volume gain’ (through water table recovery) was calculated for a series of defined ‘feature restoration lengths’ (e.g. total lengths of drain or gully), based on a 30m zone surrounding each feature multiplied by the average peat depth and the total proposed length of restoration.
Where the volume loss from instability is calculated to be less than 20% of the volume gain from restoration, the ‘Summary Form’ reports that “The benefits of restoration are likely to exceed the risks associated with landslides.” Where the volume loss is calculated to be >20% of the volume gain, the ‘Summary Form’ reports that “The impacts of potential slope instability may equal or exceed the benefits of restoration.” Where risks are Low or Negligible, the former result is reported by default.
5.4.6. Reducing risk
5.4.6.1. Approaches to reducing risk
In the event of an unacceptable risk outcome for a site, there are two primary approaches to revising the site assessment (under the assumption that the initial data inputs are best estimates rather than overly conservative judgements):
- Subdividing the restoration site: into two (or more) areas that better reflect the variability in landslide likelihood over the proposed restoration site.
- Modifying the proposed restoration techniques: to those which improve stability, or discarding approaches that reduce stability.
In relation to 1, dependent on the extent of a restoration site, there may be significant differences in slope angle, peat depth, drainage density and/or alignment, or other factors that drive the baseline landslide likelihood. Step 1 of the protocol workbook indicates the scores associated with the selected data inputs and can be used to identify which factors drive landslide likelihood.
For example, if an area of planar peat with pools is generating a high likelihood of bog burst, then avoidance of such an area enables a change of data input and a reduction in likelihood score by 1. Equally, if the site is relatively consistent in ground conditions, but a specific receptor is driving a high risk, then the area of the site close to or adjoining that receptor may be omitted from the restoration plan enabling the consequences and the associated calculated risks to be reduced.
In relation to 2, the net effect of each technique is shown in Step 2 of the protocol workbook (with effects varying according to drain orientation, for example). Modifications can be made to the proposed techniques by omission or substitution of one technique for another.
It should also be noted that some techniques have no impact on instability due to the lack of impact on ground conditions. Bare peat revegetation, spot turving and transplanting are all interpreted to have negligible effects on stability (possibly increasing it, though not quantifiably), while gully reprofiling and/or gully damming/bunding generally increase stability by shoring up gully sides and providing more tensile strength as vegetation regrows. In both cases, there is the potential for minor instability (Figure 2.3) in association with plant movements, but this should be manageable by good site practice and use of experienced contractors.
5.4.6.2. Post-mitigation landslide likelihood and residual risk
Once the baseline likelihood, post-restoration likelihood or consequence steps have been revisited, either through subdivision of the site or revisiting inputs, the protocol will update calculated risks for the restoration site, and the ‘final’ risk outcomes should be recorded on the Summary Form. At this point, a decision will be required on whether to take the site forward or whether not to restore based on calculated risks.
5.4.6.3. Decision making on restoration with respect to peat landslide risk
The purpose of the protocol is not to limit restoration but to ensure that the techniques used are applied in locations where risks associated with peat instability are low and are undertaken using methods that do not increase instability significantly. Because raising water tables is a key aim of restoration but also a key factor in reducing stability, the positive impacts of restoration must be carefully considered against potentially negative consequences.
Based on the ‘final’ risk outcomes, the following approaches are recommended:
- For sites with Negligible/Low modified (post-restoration) risks: restoration should continue as proposed and the Peatland ACTION officer should note the results of the protocol on the Summary Form and append to the project file.
- For sites with Medium modified (post-restoration) risks: the specific site receptors that are associated with these risks should be reviewed at an organisational level (i.e. by the Peatland ACTION management team), and a decision reached according to the perceived value of the restoration project and the consensus view on the reliability of the input data and value of those receptors (see below).
- For sites with High modified (post-restoration) risks: restoration involving significant groundworks should be avoided, and less intrusive works specified (e.g. revegetation of bare peat) as an alternative.
Further information to support review of Medium and High risk sites is provided below.
5.4.6.4. Reliability of data
The inputs to the baseline likelihood assessment are site averaged values for each ground condition (as noted in Section 4.2). Averaging may simultaneously under-represent the most adverse ground conditions and overstate how adverse conditions are in some of the least susceptible parts of a site. Accordingly, there may be a need to consider how representative the datasets are of the site under consideration, particularly if restoration groundworks are focused in parts of the site with relatively benign ground conditions.
Table 5.3 indicates potential sources of uncertainty in the data inputs for the ground conditions used and how these might be addressed when using the protocols.
If review of the data inputs indicates a robust case for amending selections within the protocol, then the logic for modifying the inputs should be documented and a file note generated to accompany any revised versions of the protocol sheets.
Table 5.3. Sources of uncertainty in input data
Ground conditions |
Sources of uncertainty |
---|---|
Peat depth |
Lack of site specific peat depth data (either none are available or sample points are poorly selected) Acquire site specific data where possible |
Slope angle |
Site is highly variable; high resolution site specific data are not available For blanket bogs, Google Earth ProTM and MagicMaps provide reasonably representative data, but where sites are highly variable they should be subdivided; for raised bogs, Google Earth ProTM and MagicMaps may not be sufficient and DTM purchase may be necessary |
Slope form (blanket bogs) |
Site is highly variable Choose the slope form most representative of the majority of the restoration area (at a hillslope scale, i.e. from summit to valley floor) |
Slope form (dome shape) |
Site is asymmetrical or non-uniform and doming is difficult to specify Choose the profile shape that describes the maximum elevation (dome centre) to the lowest elevation to ensure suitable conservatism |
Substrate geology |
No exposures are present during the site visit or BGS data are non-specific Select ‘Unknown’ within the protocol (which provides a reasonably conservative score) |
Peat morphology |
Site is highly variable; the extent of pipe networks is not clear from the available data Subdivide site if some morphology is high scoring (e.g. pools or pipes) to avoid over-conservatism in areas without this morphology |
Peat drainage |
Drain orientation is highly variable Subdivide site if some drainage is high scoring to avoid over-conservatism in areas with lower scoring drainage |
Forestry |
Present-day site conditions do not correspond to satellite imagery Request felling information from landowner and use satellite imagery for alignment only (not status, i.e. afforested or deforested) |
Land use |
Depth of burning unknown Undertake a site visit, if possible |
5.4.6.5. Value of the receptor
In order to enable calculation of risk, severity levels have been assumed in association with each receptor type (see Table 5.2). The severity levels do not consider the scale of the receptor or the relative value of different designations, however, in practice there may be good reasons for a specific receptor type to have differing severity from site to site.
The calculated risks generated in Step 3 are drawn from the Peat Landslide Likelihood / Adverse Consequence matrix shown within the protocol. Although the protocol cannot be edited, it is possible to calculate an alternative risk result by comparing the post-restoration likelihood output from Stage 2 with a chosen severity level. For example, if the post-restoration likelihood is ‘Moderate’ and a decision is made to modify a severity level from ‘Very High’ (e.g. for a railway line) to ‘Moderate’ (because it is on an embankment) shown in Table 5.2, then the updated severity level can be read from the ‘Adverse Consequence’ columns reproduced from the risk matrix on Figure 5.3 below. Instead of a Medium risk outcome, a Low risk outcome would result.
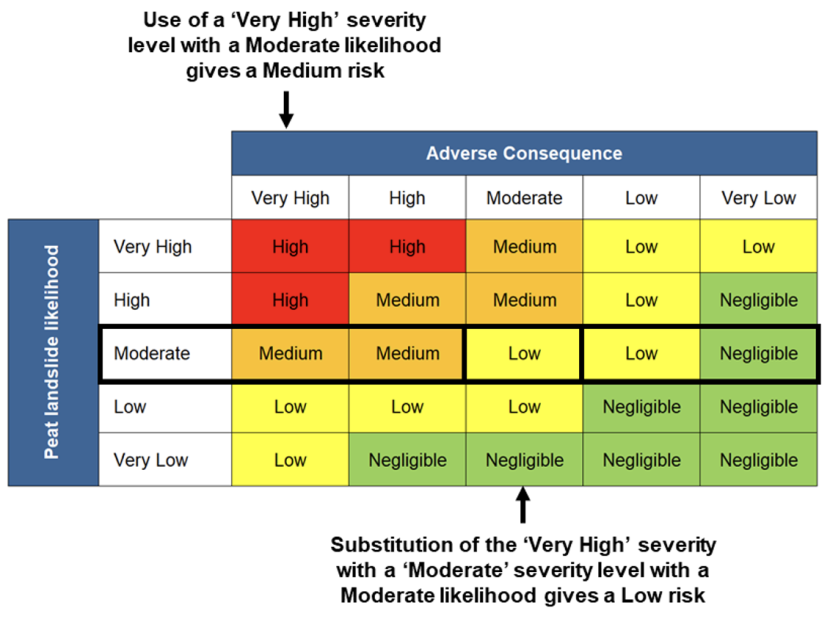
Again, it is important to document where departures from the protocol are applied. In the event that such departures are frequently required in order to facilitate restoration, there may be a need to revisit the inputs to the protocol (see Chapter 6).
5.4.6.6. Involving a third party
It is usual practice in engineering projects to undertake detailed site-specific analysis where a site indicates potential for instability. The protocols are intended to provide a high level tool based on representative data, but are not detailed assessments equivalent to those used to support engineering. In the event that a site is sufficiently complex and there are potentially significant benefits to the restoration being proposed, there may be a need to undertake a more detailed assessment of stability, more akin to that utilised for other projects in peatlands (such as wind farms). There are numerous third parties now undertaking such assessments, the majority of which are informed by and adhere to principles laid out in the Scottish Government’s (2017) Peat Landslide Hazard and Risk Assessment Best Practice Guide. It is suggested that where a ‘stalemate’ is reached for a site with significant potential benefits, advice should be sought from an appropriately qualified third party.
5.4.7. Assessing intermediate bogs
Intermediate bogs have characteristics of both raised bogs and blanket bogs and usually occur at elevations between 30m and 150m (JNCC, 1994). In common with raised bogs, they may occur as isolated areas of peat in a non-peatland landscape with their margins modified by agriculture, forestry or peat cutting. They may share morphological similarities with raised bogs, with visible doming, and where extensive, may extend onto gentle slopes and share some similarities with blanket bogs or merge into blanket bogs.
From the point of view of applying the risk-based protocols, the following is recommended:
- Where an intermediate bog is domed, has discrete margins and is located on neutrally sloping terrain or slopes <5°, the raised bog protocol should be used.
- Where an intermediate bog is draped across undulating terrain with no clear gradation into mineral soil, and where local slopes (over 10s of metres) exceed 5°, the blanket bog protocol should be used.
It is recommended that the reasoning behind the choice of protocol is documented as a file note to the application.
5.4.8. Subdividing sites
Where sites may merit subdivision in order to reassess likelihoods and/or consequences, gross differences in topography, land cover or restoration type should be used as the primary basis for differentiation. For example, if a site comprises gullying in one area and artificial drainage in another, splitting the site in two may be merited to reflect the relative influence of each ground character on both baseline likelihood and restoration impact.
Figure 5.4 shows an example landscape in which three separate land classes are highlighted. Three different landscape characters are shown:
- Gentle to moderately sloping terrain with occasional watercourses (with likely shallow peat in the lower slopes and deeper peat towards the slope crests) – peat slides may be the most likely failure mechanism.
- Plateaux with pool systems (with likely deep peat and high moisture content between the pools) – bog bursts may be the most likely failure mechanism.
- Moderate to steep slopes (with likely thin peat, mineral soil or rock outcrops) – peaty debris slides may be the most likely failure mechanism.
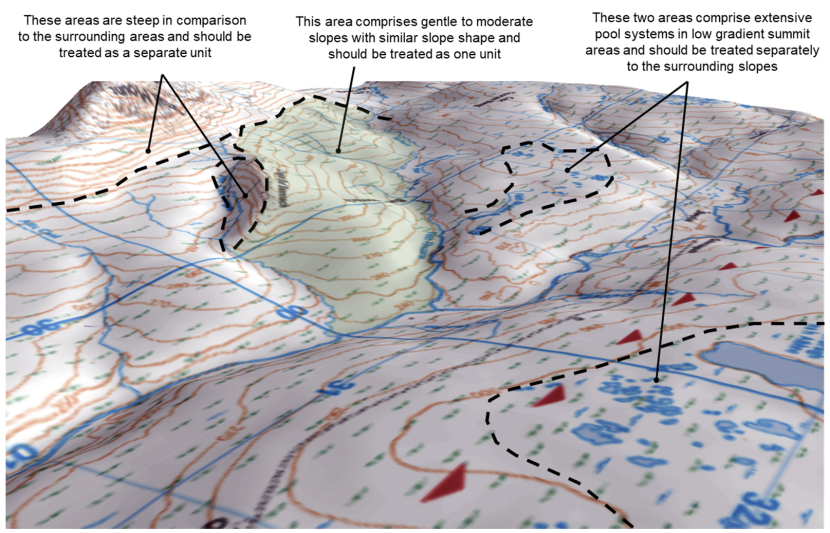
An indicative restoration project area is shown shaded green. When looking for evidence of instability on adjacent hillsides (in order to answer Q1 in Step 1 of the protocols), a “buffer” distance of c. 5km from the site boundaries is recommended. While this may seem a significant distance, peat instability is comparatively rare, and the presence of a failure within this distance may indicate local geological, micro-climatic or peatland characteristics that pre-dispose the wider area to instability.
This is supported by many of the peat landslide clusters: 20+ landslides on Cuilcagh Mountain occurred within 7km of one another, c. 10 failures at Slieve Rushen occurred within 4km of one another, 5 failures at Slievenakilla occurred within 4km of each other, the two ridges at Straduff with over 16 failures occurred within 7km of each other, 16 failures at Pollatomish occurred within 2km of each other and the 20+ failures at Channerwick / Cunningsburgh occurred within 5km of one another and 8 failures in the North Pennines occurred within 3.5km of one another.
A further basis for subdividing sites would be if there were pronounced differences in exposure of receptors, which is as relevant to the risk calculation as the likelihood. Examples might include:
- Restoration works undertaken in an area that drains to two different catchments, where one of the catchments has a designated watercourse and one does not.
- Works undertaken in an area in which one hillside and the adjacent footslopes / valley floor are under different ownership to the rest of the land in which the work is being undertaken.
- Works undertaken across an area where part of the site (within credible runout distance) is traversed by overhead line infrastructure, but a majority of the site is away from any potential impact on this receptor.
A summary sheet within each protocol gives users an opportunity to comment on how receptors are addressed within each protocol run, if the considerations have become a critical part of the risk assessment process.
6. Knowledge gaps and refinement of the protocol
6.1. Knowledge gaps and uncertainties
The approach to assessing landslide risks in relation to peatland restoration has drawn on a significant body of knowledge of peat mass movements, peatlands as soils and hydrological systems and of restoration practice. Many aspects of the approach to assessing peat landslide likelihood have been drawn from relatively well established good practice currently being applied in relation to upland developments for renewable energy. Nevertheless, there remain a number of uncertainties which should be considered when evaluating the merits of restoration schemes in sensitive locations. These are summarised below.
6.1.1. Uncertainties in the geotechnical behaviour of peat
As noted in Section 4.3.2, there remain considerable uncertainties in the understanding of the geotechnical behaviour of peat. This includes defining representative basic index properties (such as bulk density) and understanding their variability and significance. Establishing reliable strength parameters for drained and undrained conditions is known to be fraught with difficulty. The ratio between intracellular and intercellular water and its impact on pore pressure is also poorly understood. It is for these reasons that the qualitative, terrain based susceptibility approach is the primary mode of assessment for landslide likelihood (rather than Factor of Safety).
Uncertainty reduction: this uncertainty requires proactive engagement from geotechnical professionals and practitioners working within peatlands to better understand how peat behaves as a soil, and how it responds (in bulk terms) to groundworks such as water table adjustments, excavations, loading and remoulding (e.g. by handling).
6.1.2. Uncertainties in the medium to long term response of peatlands to restoration measures
While this study has enabled an initial view of the impacts of restoration on ground stability, many schemes are still being planned or implemented and there is a small dataset of post-restoration sites from which to collect evidence about responses to groundworks. While monitoring can be undertaken using increasingly resolute Google Earth satellite imagery, the most useful information could be realised by strategic monitoring of representative sites.
Uncertainty reduction: Section 6.3 provides more specific recommendations in relation to monitoring.
6.1.3. Limitations
The approaches adopted within this study are to an extent limited by the requirement to produce something accessible to personnel without geoscience training to evaluate a geotechnical problem. This means:
- The approach is applied over broad spatial areas: (e.g. hillsides) and therefore is not spatially resolute – it is therefore applicable at ‘site scales’ and is not appropriate for local stability analysis (even if the protocol data are input at local scale). Local stability analysis (if required) should be undertake by appropriately trained specialists.
- The approach simplifies ground conditions: both spatially (as above) and in terms of classifying ground conditions within predefined ranges (e.g. peat depths from 0.5-1.5m). This reflects the use of ‘site average’ conditions, which must be broad enough to encompass the most representative ground conditions while discrete enough to enable differentiation between sites.
- The approach takes no consideration of site practice: it is assumed that all restoration practitioners / contractors apply safe systems of work and employ good practice during groundworks as defined in the SNH Peatland ACTION Technical Compendium, Draft v6; SNH, May 2019. The assessment of impacts of each technique has been based on the methods described in this compendium and later versions have not been assessed. Further discussion of evolving techniques is provided in Section 6.2.
- Understanding of post-restoration impacts is limited by lack of monitoring: although satellite imagery has been used to review a proportion of the sites that have undergone restoration, many sites had no data available post-groundworks, and at present, unless already part of a research site (e.g. the Forsinard Flows Nature Reserve or research sites being worked by Forestry and Land Scotland), the long term impacts of restoration are not being widely monitored. Therefore, the assumption that restoration has a minimal negative impact on peat stability lacks data to support it.
6.2. Evolving site practice
At the time of writing, the Scottish Government announced £250m to support peatland restoration in Scotland over a ten-year period (from 2020). As a result, it is likely that the techniques considered in this study will continue to be applied and improved and new techniques will be developed.
The protocols have been designed to enable addition of new techniques and modification of their impacts (e.g. from a net neutral impact on stability to a net increase in stability) by amendments to a locked down worksheet within each protocol. Each technique has been considered both qualitatively and quantitatively and it is a relatively minor workflow to address changes in technique or add new techniques to the protocol. Recommendations in this regard are provided in Section 6.4.
6.3. Monitoring restored sites
6.3.1. The need for monitoring
Site monitoring is important for two reasons: i) it enables the physical benefits of restoration work to be assessed and provides justification for restoration budgets, ii) it enables different restoration techniques to be compared in order to identify the best return on expenditure, and iii) it enables other impacts of restoration (beyond those related to habitat) to be evaluated, including any adverse impacts on ground stability.
6.3.2. Monitoring for stability
Monitoring of ground stability post-restoration can be undertaken using either remotely sensed data (such as periodic satellite or aerial imagery available on Google Earth Pro or from data re-sellers) or by direct site observation and/or monitoring.
6.3.2.1. Monitoring using satellite / aerial imagery
The value of remotely sensed data has already been demonstrated in Section 3.1, and it is recommended that routine review of restored sites is undertaken by the Peatland ACTION data team using information provided in this report to help identify features that might be associated with instability. In the event that potential instability is identified, there may be merit in the area Peatland ACTION officer visiting the site to review ground conditions. If instability is confirmed, site conditions should be recorded and a note added to the Instability Record in Annex 4 (see Section 6.3.2.2 below)
6.3.2.2. Monitoring by direct site observation and/or monitoring
Direct site observations may be required where instability develops during restoration groundworks. For example, if small-scale instability is observed (such as formation of a tension crack), the following approaches can be used:
- Fixed point photography: photography of areas of concern showing ground conditions over multiple visits. A clear reference marker is required on-site from which to take the photograph, as is the field of view (bearing) – ideally, the same camera should be used on each visit. Changes in ground conditions should be noted from one visit to the next.
- Ground movement markers: vertical rods inserted upslope and downslope of a crack or area of subsidence can be used to identify displacement (e.g. if rods become further apart, then the peat mass is translating downslope; if rods tilt, the base may be caught in the substrate and indicate translate or there may be a rotational component of ground displacement). Movement markers may also be inserted into the ground in lines, and deviations from the line used to identify more subtle rates of change.
Both of these approaches are low cost, easy to install and require no special training to undertake.
If ground movements occur over multiple site visits, there may be merit in seeking third party advice to ensure that minor ground displacements to do not propagate into larger-scale and more catastrophic failure. However, it should be noted that displacements often relieve short term stresses and may be ‘one offs’, so it is advisable to monitor a site and be sure that change is continuing before seeking such advice (particularly if there receptors are of relatively low value).
6.3.3. Monitoring the effectiveness of restoration
It is outside the scope of this report to consider restoration monitoring in relation to habitat and hydrology, however, in the case of the latter, records of rising water tables may help provide context to instability, should it occur at a later date. The hydrological effectiveness of restoration need not require the use of dipwells or piezometers to provide a continuous record, and their installation requires specialist knowledge, cost and data gathering. Instead, change in species composition in restored areas may provide a proxy for water table changes if there is an observable change from drier to wetter species. This form of monitoring requires some knowledge of peatland habitat by PA officers.
UAV (unmanned aerial vehicles) or drone surveys provide opportunities to survey large areas at high resolution at a time of a practitioner or land manager’s choosing. The level of detail is may be sufficient to identify gross changes in habitat composition without the need to walk over an extensive site area. Furthermore, UAVs also provide a good opportunity to collect site-specific data that could be used to inform protocol completion (including slope, geomorphology and drainage information). UAV technology continues to develop and data becomes cheaper to acquire, easier to process and operational under a wider range of windspeeds (which can be a significant constraint in remote uplands).
6.4. Refining the protocol
6.4.1. Reasons for refinement
There are three primary reasons to consider refinement to the protocols:
- The protocol data inputs lead to an excessive number of ‘unrestorable’ sites: due either to overconservative assessment of landslide likelihood or overstatement of consequences for receptors. Whether this is the case will only become clear once the protocol has been widely used by Peatland ACTION officers, however, it should be noted that the approach is based on an established methodology that typically predicts Very Low to Low likelihoods of instability over most sites, other than in areas of moderate slope and moderate depth peat (where instability features are often observed). An initial round of calibration has been undertaken following 12 months of use by Peatland ACTION officers in a range of settings, and further details are provided in section 6.5.
- Post-restoration monitoring indicates that some techniques are commonly associated with instability: it has been noted that monitoring to-date has been relatively limited – it is possible that as restoration is applied over more sites and larger areas, some techniques become associated with instability. If this is the case, then the reasons for instability should be investigated and the ‘impacts on stability’ considered in Section 4.4 should be revisited.
- New restoration techniques are developed or existing techniques are modified: the protocols consider all techniques listed in v6 of the Technical Compendium. However, at the time of writing, v8 had been released and v9 was in progress. If techniques are omitted from Step 2 of the two protocols, then their effects cannot be considered alongside other more established techniques.
In the case of 3. above, it is accepted that many techniques are experimental in their early stages of application, and it may require several site trials before a technique is brought into the Peatland ACTION toolbox. The most appropriate point at which to update the protocols to reflect a new technique is once the technique has been considered suitable to widespread rollout (without supervision by Peatland ACTION officers).
6.4.2. Implementing changes
The protocol workbooks are locked to prevent unanticipated edits to the calculations that deliver the risk outcomes. Edits to these equations and the data inputs should be undertaken by those familiar with the approaches used to calculate likelihood, consequence and risk.
A change log is provided at the front of this report to illustrate where updates to the text and protocols have been made. Annex 5 provides a record sheet within which to document the reasons for changes, the adjustments made to the text and modifications to the protocol.
6.5. Protocol calibration
Following the first twelve months of use, a range of sites were assessed as part of a calibration exercise for both the blanket bog and raised bog protocols. The purpose of calibration was to determine:
- Whether calculated pre-restoration likelihoods reflected observed site conditions.
- Whether calculated risks were proportionate to the perceived value of receptors and their connectivity to potential landslide source areas.
- Whether the protocols were sufficiently clear to enable ‘standalone’ use without additional technical support by the report authors.
The review comprised detailed inspection of 10 raised bog sites and 12 blanket bog sites for which protocols had previously been completed by Peatland ACTION officers, with a subset in each setting having experienced pre-restoration peat landslides. The review included:
- Inspection of satellite imagery for evidence of past instability or incipient failures.
- Generation of peat depth models using site-specific probe data to validate peat depth inputs.
- Review and verification / validation of all data inputs by users to determine validity of pre-restoration likelihood inputs.
- Inspection of receptor type, qualifying interest and location to determine validity of consequence assessments.
Based on this review:
- Data inputs by Peatland ACTION officers were a good representation of site conditions (noting that there had been minimal need to provide support to Peatland ACTION officers in completing the protocols during the 12 months).
- Some blanket bogs, particularly those with drains aligned oblique to slope (or contour aligned) in typical peat depth and slope angle ranges were excessively disposed towards Moderate pre-restoration likelihoods given the observed site features.
- The slope form criterion had insufficient options to reflect the undulating topography associated with peatlands in areas with strong bedrock control (ultimately leading to excessive slope form scores for these settings, and in turn higher pre-restoration likelihoods).
- The consequence assessment lacked granularity and steer relative to the pre-restoration and post-restoration likelihood inputs.
- For raised bogs, the protocol findings provided a very good match between pre-restoration likelihoods and observed instability features.
Given these findings, a number of changes were made to the blanket bog protocol to reduce the (over)conservatism for sites in some settings. Adjustments were also made to a number of data inputs to provide additional detail and options. The post-restoration inputs were updated to separate drain blocking effects from forestry restoration effects. The consequence and risk inputs were updated to provide better steer on judgements. The post-restoration and consequence and risk inputs for the blanket bog and raised bog protocols are the same, and therefore the changes for the blanket bog protocol were carried across to a revised version of the raised bog protocol.
7. References
Alderson, D.M., Evans, M.G., Shuttleworth, E.L., Pilkington, M., Spencer, T., Walker, J. and Allott, T.E.H. 2019. Trajectories of ecosystem change in restored blanket peatlands. Science of the Total Environment, 665, 785-796.
Armstrong, A., Holden, J., Kay, P., Foulger, M., Gledhill, S., McDonald, A.T. and Walker, A. 2009. Drain-blocking techniques on blanket peat: a framework for best practice. Journal of Environmental Management, 90, 3512-3519.
Bonn, A., Allott, T., Evans, M., Joosten, H. and Stoneman, R. 2016. Peatland restoration and ecosystem services: science, policy and practice. Cambridge University Press. 493p.
Bowes, D.R. 1960. A bog-burst in the Isle of Lewis. Scottish Geographical Journal. 76, 21-23.
Boylan, N., Jennings, P. and Long, M. 2008. Peat slope failure in Ireland. Quarterly Journal of Engineering Geology, 41, 93-108.
Boylan, N. and Long, M. 2011. In situ strength characterisation of peat and organic soil using full-flow penetrometers. Canadian Geotechnical Journal, 48(7), 1085-1099.
Boylan, N. and Long, M. 2014. Evaluation of peat strength for stability assessments. Geotechnical Engineering, 167, 422-430.
Carling, P.A. 1986. Peat slides in Teesdale and Weardale, Northern Pennines, July 1983: description and failure mechanisms. Earth Surface Processes and Landforms, 11, 193-206.
Chapman, S., Hester, A., Irvine, J. and Pakeman, R. 2017. Muirburn, peatland and peat soils – an evidence assessment of impact. James Hutton Institute, 24p.
Creighton, R. 2006. ed. Landslides in Ireland. Geological Society of Ireland, Irish Landslides Working Group, 125p
Creighton, R. and Verbruggen, K. 2003. Geological Report on the Pollatomish Landslide Area, Co. Mayo. Geological Survey of Ireland, 13p.
Cullen, C. 2011. Peat stability – minimising risks by design. Presentation at SEAI Wind Energy Conference 2011, 45p.
Dykes, A.P. and Kirk, K.J. 2001. Initiation of a multiple peat slide on Cuilcagh Mountain, Northern Ireland. Earth Surface Processes and Landforms, 26, 395-408.
Dykes, A. and Warburton, J. 2007a. Mass movements in peat: A formal classification scheme. Geomorphology, 86, 73-93.
Dykes, A. and Warburton, J. 2007b. Significance of geomorphological and subsurface drainage controls on failures of peat-covered hillslopes triggered by extreme rainfall. Earth Surface Processes and Landforms, 32, 1841-1862.
Dykes, A.P. 2008. Tensile strength of peat: Laboratory measurement and role in Irish blanket bog failures. Landslides 5(4), 417-429. doi: 10.1007/s10346-008-0136-1.
Dykes, A. and Warburton, J. 2008. Failure of peat-covered hillslopes at Dooncarton Mountain, Co. Mayo, Ireland: analysis of topographic and geotechnical factors. Catena, 72, 129-145.
Evans, M.G. and Warburton, J. 2007. Geomorphology of Upland Peat: Erosion, Form and Landscape Change, Blackwell Publishing, 262p.
Farrell, E.R. and Hebib, S. 1998. The determination of the geotechnical parameters of organic soils, Proceedings of International Symposium on Problematic Soils, IS-TOHOKU 98, Sendai, 1998, Japan, 33-36.
Feehan, J. and O’Donovan, G. 1996. The bogs of Ireland: an introduction to the natural, cultural and industrial heritage of Irish peatlands. University College Dublin, 518p.
Flaherty, R. 2016. Man dies in suspected landslide at wind farm in Co Sligo. Irish Times, 13/12/2013.
Forest and Land Scotland. 2019. Mossband Monitoring Visit. Unpublished Report. 16p.
Forestry Commission Scotland. 2015. Deciding future management options for afforested deep peatland: practice guide. Forestry Commission Scotland, 25p.
Google. 2020. Google Earth Pro.
Hebib, S. 2001. Experimental investigation of the stabilisation of Irish peat, unpublished PhD thesis, Trinity College Dublin.
Henderson, S. 2005. Effects of a landslide on the shellfish catches and water quality in Shetland. Fisheries Development Note No. 19, North Atlantic Fisheries College.
Hobbs, N.B. 1986. Mire morphology and the properties and behaviour of some British and foreign peats. Quarterly Journal of Engineering Geology, London, 1986, 19, 7–80.
Holden, J. 2005. Controls of soil pipe frequency in upland blanket peat. Journal of Geophysical Research, 110, 11p.
Holden, J. and Burt, T.P. 2003. Runoff production in blanket peat covered catchments. Water Resources Research, 39(7), 1191.
Holden, J., Green, S.M., Baird, A.J., Grayson, R.P., Dooling, G.P., Chapman, P.J., Evans, C.D., Peacock, M. and Swindles, G. 2017. The impact of ditch blocking on the hydrological functioning of blanket peatlands. Hydrological Processes, 31, 525-539.
Huat, B.B.K., Prasad, A., Asadi, A. and Kazemian, S. 2014. Geotechnics of organic soils and peat. Balkema, 269p.
James Hutton Institute. 2020. Peat surveys: peat deposits.
JNCC. 1994. Guidelines for the Selection of Biological SSSIs. Part 2: Detailed Guidelines for Habitats and Species Groups. Chapter 8 Bogs. JNCC, Peterborough.
Lantschner, L., McMullen, A. and MacDougall, K. 2011. Restoration Techniques Using Peat Spoil from Construction Works. EnviroCentre, 41p.
Lee, E.M. and Jones, D. 2013. Landslide Risk Assessment, 2nd edition. ICE Publishing, 518p.
Lindsay, R.A. and Bragg, O. 2005. Wind farms and blanket peat – a report on the Derrybrien bog slide, 2nd edition. Ireland Derrybrien Development Cooperative Ltd. 149p.
Lindsay, R.A. and Immirzi, P. 1996. An inventory of lowland raised bogs in Great Britain. Scottish Natural Heritage.
Lindsay, R.A. 1995. Bogs: the ecology, classification and conservation of ombotrophic mires. Scottish Natural Heritage.
Long, M. 2005. Review of peat strength, peat characterisation and constitutive modelling of peat with reference to landslides. Studia Geotechnica et Mechanica, XXVII, 3-4, 67-88.
Long, M., Trafford, A. and Donohue, S. 2014. Investigation of failures in Irish raised bogs. Landslides, 11(4), 733-743.
McIntosh, B. 2020. BGS Geoheritage – images from the collections: Black Moss, Dinnet, Aberdeenshire. Kieselguhr or diatomite works 1917.
Mills, A.J. 2002. Peat slides: Morphology, Mechanisms and Recovery, unpublished PhD thesis, University of Durham.
Mills, A.J., Moore, R., Carey, J.M. and Trinder, S.K. 2007. Recent landslide impacts in Scotland: possible evidence of climate change? In: McInnes, R. et al., eds. Landslides and Climate Change: Challenges and Solutions, Proceedings of Conference, Isle of Wight.
NatureScot. 2022. Peatland ACTION – Technical Compendium.
Page, S.E., Siegert, F., Rieley, J.O., Boehm, H-D.V., Jaya, A. and Limin, S. 2002. The amount of carbon released from peat and forest fires in Indonesia during 1997. Nature, 420, 61-65.
Peatland ACTION. 2015. Greenhead Moss, hydrology and peat depth survey. North Lanarkshire Council. 19p.
Peatland ACTION. 2018. Innovation in peatland restoration with Peatland ACTION. Workshop Presentation, 97p.
Peatland ACTION. 2019. Dam installation techniques – peat and plastic dams. Guidance for land managers, 24p.
Pickett, B. 2018. Machines on the moss – turning purple to green.
RSK. 2018. Engineer’s Quick Reference Guide for Ground Investigation, 56p.
Scottish Government. 2017. Peat Landslide Hazard and Risk Assessments, Best Practice Guide for Proposed Electricity Generation Developments (Second Edition). Scottish Government, 84p.
Scottish Government. 2016. Getting The Best From Our Land, A Land Use Strategy for Scotland. Scottish Government, 42p.
Scottish Government. 2011. Wind Farm Developments on Peatlands. Scottish Government, 1p.
Scottish Government. 2009. Management of Carbon-Rich Soils – Overview and Discussion Paper.
Scottish Government, Scottish Natural Heritage, SEPA. 2017. Peatland Survey. Guidance on Developments on Peatland, on-line version only, 18p.
Scottish Lowlands Forest District. 2014. Murdostoun and Mossband Forest Plan (2015-2025) Unpublished Report, 41p.
Scottish Natural Heritage. 2015a. National Peatland Plan.
Scottish Natural Heritage. 2015b. Constructed tracks in the Scottish Uplands, 2nd Edition. 147p.
Taylor, E. 2019. Restoration Techniques, Peatland ACTION Technical Compendium (Draft v6). Internal Report, May 2019, 48p.
Scottish Renewables and Scottish Environmental Protection Agency. 2012. Developments on Peatland: Guidance on the assessment of peat volumes, reuse of excavated peat and the minimisation of waste. Version 1, January 2012. SR and SEPA Joint Publication, 23p.
SEPA. 2010. SEPA Regulatory Position Statement – Developments on Peat. National Waste Policy Unit, SEPA, 4p.
The Shetland Times. 2015. Mid Kame landslip on proposed windfarm site.
Shuttleworth, E.L., Evans, M.G., Pilkington, M., Spencer, T., Walker, J., Milledge, D. and Allott T.E.H. 2018. Restoration of blanket peat moorland delays stormflow from hillslopes and reduces peak discharge. Journal of Hydrology, X(2), 14p.
Sloan, T.J., Payne, R.J., Anderson, A.R., Gilbert, P., Mauquoy, D., Newton, A.J. and Andersen, R. 2019. Ground surface subsidence in an afforested peatland fifty years after drainage and planting. Mires and Peat, 23, 1-12
Stewart, A.J. and Lance, A.N. 1983. Moor-draining – a review of impacts on land-use. Journal of Environmental Management, 17, 81-99.
Warburton, J., Holden, J. and Mills, A.J. 2004. Hydrological controls of surficial mass movements in peat. Earth Science Reviews, 67, 139-156.
Warburton, J., Higgitt, D. and Mills, A.J. 2003. Anatomy of a Pennine peat slide, Northern England. Earth Surface Processes and Landforms, 28, 457-473.
Warburton, J. 2015. Peat landslides. In: Shroder, J.F. and Davies, T. eds. Landslides, Hazards, Risks and Disasters, Elsevier, 159-190.